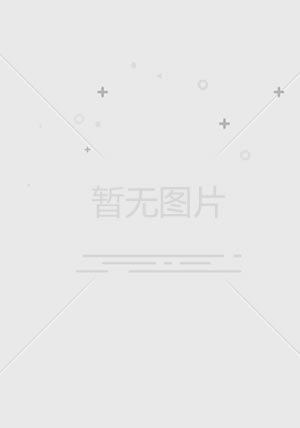
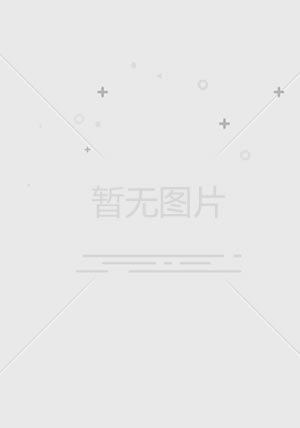
lehninger principles of biochemistry fourth editionPDF电子书下载
- 电子书积分:20 积分如何计算积分?
- 作 者:
- 出 版 社:
- 出版年份:2222
- ISBN:
- 页数:0 页
1 The Foundations of Biochemistry 1
1.1 Cellular Foundations 3
Cells Are the Structural and Functional Units of All Living Organisms 3
Cellular Dimensions Are Limited by Oxygen Diffusion 4
There Are Three Distinct Domains of Life 4
Escherichia coli Is the Most-Studied Prokaryotic Cell 5
Eukaryotic Cells Have a Variety of Membranous Organelles,Which Can Be Isolated for Study 6
The Cytoplasm Is Organized by the Cytoskeleton and Is Highly Dynamic 9
Cells Build Supramolecular Structures 10
In Vitro Studies May Overlook Important Interactions among Molecules 11
1.2 Chemical Foundations 12
Biomolecules Are Compounds of Carbon with a Variety of Functional Groups 13
Cells Contain a Universal Set of Small Molecules 14
Macromolecules Are the Major Constituents of Cells 15
Box 1-1 Molecular Weight, Molecular Mass, and Their Correct Units 15
Three-Dimensional Structure Is Described by Configuration and Conformation 16
Box 1-2 Louis Pasteur and Optical Activlty: In Vino, Veritas 19
Interactions between Biomolecules Are Stereospecific 20
1.3 Physical Foundations 21
Living Organisms Exist in a Dynamic Steady State, Never at Equilibrium with Their Surroundings 21
Organisms Transform Energy and Matter from Their Surroundings 22
The Flow of Electrons Provides Energy for Organisms 22
Creating and Maintaining Order Requires Work and Energy 23
Energy Coupling Links Reactions in Biology 23
Box 1-3 Entropy: The Advantages of Belng Disorganized 24
Keq and △G Are Measures of a Reaction's Tendency to Proceed Spontaneously 26
Enzymes Promote Sequences of Chemical Reactions 26
Metabolism Is Regulated to Achieve Balance and Economy 27
1.4 Genetic Foundations 28
Genetic Continuity Is Vested in Single DNA Molecules 29
The Structure of DNA Allows for Its Replication and Repair with Near-Perfect Fidelity 29
The Linear Sequence in DNA Encodes Proteins with Three-Dimensional Structures 29
1.5 Evolutionary Foundations 31
Changes in the Hereditary Instructions Allow Evolution 31
Biomolecules First Arose by Chemical Evolution 32
Chemical Evolution Can Be Simulated in the Laboratory 32
RNA or Related Precursors May Have Been the First Genes and Catalysts 32
Biological Evolution Began More Than Three and a Half Billion Years Ago 34
The First Cell Was Probably a Chemoheterotroph 34
Eukaryotic Cells Evolved from Prokaryotes in Several Stages 34
Molecular Anatomy Reveals Evolutionary Relationships 36
Functional Genomics Shows the Allocations of Genes to Specific Cellular Processes 38
Genomic Comparisons Will Have Increasing Importance in Human Biology and Medicine 38
Ⅰ STRUCTURE AND CATALYSIS 45
2 Water 47
2.1 Weak Interactions in Aqueous Systems 47
Hydrogen Bonding Gives Water Its Unusual Properties 47
Water Forms Hydrogen Bonds with Polar Solutes 49
Water Interacts Electrostatically with Charged Solutes 50
Entropy Increases as Crystalline Substances Dissolve 51
Nonpolar Gases Are Poorly Soluble in Water 52
Nonpolar Compounds Force Energetically Unfavorable Changes in the Structure of Water 52
van der Waals Interactions Are Weak Interatomic Attractions 54
Weak Interactions Are Crucial to Macromolecular Structure and Function 54
Solutes Affect the Colligative Properties of Aqueous Solutions 56
Box 2-1 Touch Response in Plants: An Osmotic Event 59
2.2 Ionization of Water, Weak Acids, and Weak Bases 60
Pure Water Is Slightly Ionized 60
The Ionization of Water Is Expressed by an Equilibrium Constant 61
The pH Scale Designates the H+ and OH-Concentrations 61
Box 2-2 The Ion Product of Water: Two Illustrative Problems 62
Weak Acids and Bases Have Characteristic Dissociation Constants 63
Titration Curves Reveal the pKa of Weak Acids 64
2.3 Buffering against pH Changes in Biological Systems 65
Buffers Are Mixtures of Weak Acids and Their Conjugate Bases 66
A Simple Expression Relates pH, pKa, and Buffer Concentration 66
Weak Acids or Bases Buffer Cells and Tissues against pH Changes 67
Box 2-3 Solving Problems Using the Henderson-Hasseibalch Equation 67
Box 2-4 Blood, Lungs, and Buffer: The Bicarbonate Buffer System 69
2.4 Water as a Reactant 69
2.5 The Fitness of the Aqueous Environment for Living Organisms 70
3 Amino Acids, Peptides, and Proteins 75
3.1 Amino Acids 75
Amino Acids Share Common Structural Features 76
The Amino Acid Residues in Proteins Are L Stereoisomers 77
Amino Acids Can Be Classified by R Group 78
Uncommon Amino Acids Also Have Important Functions 80
Amino Acids Can Act as Acids and Bases 81
Box 3-1 Absorption of Light by Molecules: The Lambert-Beer Law 82
Amino Acids Have Characteristic Titration Curves 82
Titration Curves Predict the Electric Charge of Amino Acids 84
Amino Acids Differ in Their Acid-Base Properties 84
3.2 Peptides and Proteins 85
Peptides Are Chains of Amino Acids 85
Peptides Can Be Distinguished by Their Ionization Behavior 86
Biologically Active Peptides and Polypeptides Occur in a Vast Range of Sizes 86
Polypeptides Have Characteristic Amino Acid Compositions 87
Some Proteins Contain Chemical Groups Other Than Amino Acids 88
There Are Several Levels of Protein Structure 88
3.3 Working with Proteins 89
Proteins Can Be Separated and Purified 89
Proteins Can Be Separated and Characterized by Electrophoresis 92
Unseparated Proteins Can Be Quantified 94
3.4 The Covalent Structure of Proteins 96
The Function of a Protein Depends on Its Amino Acid Sequence 96
The Amino Acid Sequences of Millions of Proteins Have Been Determined 96
Short Polypeptides Are Sequenced Using Automated Procedures 97
Large Proteins Must Be Sequenced in Smaller Segments 99
Amino Acid Sequences Can Also Be Deduced by Other Methods 100
Box 3-2 Investigating Proteins with Mass Spectrometry 102
Small Peptides and Proteins Can Be Chemically Synthesized 104
Amino Acid Sequences Provide Important Biochemical Information 106
3.5 Protein Sequences and Evolution 106
Protein Sequences Can Elucidate the History of Life on Earth 107
4 The Three-Dimensional Structure of Proteins 116
4.1 Overview of Protein Structure 116
A Protein's Conformation Is Stabilized Largely by Weak Interactions 117
The Peptide Bond Is Rigid and Planar 118
4.2 Protein Secondary Structure 120
The α Helix Is a Common Protein Secondary Structure 120
Amino Acid Sequence Affects α Helix Stability 121
Box 4-1 Knowing the Right Hand from the Left 122
The β Conformation Organizes Polypeptide Chains into Sheets 123
β Turns Are Common in Proteins 123
Common Secondary Structures Have Characteristic Bond Angles and Amino Acid Content 124
4.3 Protein Tertiary and Quaternary Structures 125
Fibrous Proteins Are Adapted for a Structural Function 126
Box 4-2 Permanent Waving Is Biochemical Engineering 127
Structural Diversity Reflects Functional Diversity in Globular Proteins 129
Box 4-3 Why Sailors, Explorers, and College Students Should Eat Their Fresh Fruits and Vegetables 130
Myoglobin Provided Early Clues about the Complexity of Globular Protein Structure 132
Globular Proteins Have a Variety of Tertiary Structures 134
Box 4-4 Methods for Determining the Three-Dimensional Structure of a Protein 136
Analysis of Many Globular Proteins Reveals Common Structural Patterns 138
Protein Motifs Are the Basis for Protein Structural Classification 141
Protein Quaternary Structures Range from Simple Dimers to Large Complexes 144
There Are Limits to the Size of Proteins 146
4.4 Protein Denaturation and Folding 147
Loss of Protein Structure Results in Loss of Function 147
Amino Acid Sequence Determines Tertiary Structure 148
Polypeptides Fold Rapidly by a Stepwise Process 148
Box 4-5 Death by Misfolding: The Prion Diseases 150
Some Proteins Undergo Assisted Folding 151
5 Protein Function 157
5.1 Reversible Binding of a Protein to a Ligand:Oxygen-Binding Proteins 158
Oxygen Can Be Bound to a Heme Prosthetic Group 158
Myoglobin Has a Single Binding Site for Oxygen 159
Protein-Ligand Interactions Can Be Described Quantitatively 160
Protein Structure Affects How Ligands Bind 162
Oxygen Is Transported in Blood by Hemoglobin 162
Hemoglobin Subunits Are Structurally Similar to Myoglobin 163
Hemoglobin Undergoes a Structural Change on Binding Oxygen 164
Hemoglobin Binds Oxygen Cooperatively 164
Cooperative Ligand Binding Can Be Described Quantitatively 167
Two Models Suggest Mechanisms for Cooperative Binding 167
Box 5-1 Carbon Monoxide: A Stealthy Killer 168
Hemoglobin Also Transports H+ and CO2 170
Oxygen Binding to Hemoglobin Is Regulated by 2,3-Bisphosphoglycerate 171
Sickle-Cell Anemia Is a Molecular Disease of Hemoglobin 172
5.2 Complementary Interactions between Proteins and Ligands: The Immune System and Immunoglobulins 174
The Immune Response Features a Specialized Array of Cells and Proteins 175
Self Is Distinguished from Nonself by the Display of Peptides on Cell Surfaces 176
Antibodies Have Two Identical Antigen-Binding Sites 178
Antibodies Bind Tightly and Specifically to Antigen 180
The Antibody-Antigen Interaction Is the Basis for a Variety of Important Analytical Procedures 180
5.3 Protein Interactions Modulated by Chemical Energy:Actin, Myosin, and Molecular Motors 182
The Major Proteins of Muscle Are Myosin and Actin 182
Additional Proteins Organize the Thin and Thick Filaments into Ordered Structures 184
Myosin Thick Filaments Slide along Actin Thin Filaments 185
6 Enzymes 190
6.1 An Introduction to Enzymes 191
Most Enzymes Are Proteins 191
Enzymes Are Classified by the Reactions They Catalyze 192
6.2 How Enzymes Work 193
Enzymes Affect Reaction Rates, Not Equilibria 193
Reaction Rates and Equilibria Have Precise Thermodynamic Definitions 195
A Few Principles Explain the Catalytic Power and Specificity of Enzymes 196
Weak Interactions between Enzyme and Substrate Are Optimized in the Transition State 196
Binding Energy Contributes to Reaction Specificity and Catalysis 198
Specific Catalytic Groups Contribute to Catalysis 200
6.3 Enzyme Kinetics As an Approach to Understanding Mechanism 202
Substrate Concentration Affects the Rate of Enzyme-Catalyzed Reactions 202
The Relationship between Substrate Concentration and Reaction Rate Can Be Expressed Quantitatively 203
Kinetic Parameters Are Used to Compare Enzyme Activities 205
Box 6-1 Transformations of the Michaelis-Menten Equation: The Double Reciprocal Plot 206
Many Enzymes Catalyze Reactions with Two or More Substrates 207
Pre-Steady State Kinetics Can Provide Evidence for Specific Reaction Steps 208
Enzymes Are Subject to Reversible or Irreversible Inhibition 209
Box 6-2 Kinetic Tests for Determining Inhibition Mechanisms 210
Enzyme Activity Depends on pH 212
6.4 Examples of Enzymatic Reactions 213
The Chymotrypsin Mechanism Involves Acylation and Deacylation of a Ser Residue 213
Hexokinase Undergoes Induced Fit on Substrate Binding 218
The Enolase Reaction Mechanism Requires Metal Ions 219
Box 6-3 Evidence for Enzyme-Transition State Complementarity 220
Lysozyme Uses Two Successive Nucleophilic Displacement Reactions 222
6.5 Regulatory Enzymes 225
Allosteric Enzymes Undergo Conformational Changes in Response to Modulator Binding 225
In Many Pathways a Regulated Step Is Catalyzed by an Allosteric Enzyme 226
The Kinetic Properties of Allosteric Enzymes Diverge from Michaelis-Menten Behavior 227
Some Regulatory Enzymes Undergo Reversible Covalent Modification 228
Phosphoryl Groups Affect the Structure and Catalytic Activity of Proteins 228
Multiple Phosphorylations Allow Exquisite Regulatory Control 230
Some Enzymes and Other Proteins Are Regulated by Proteolytic Cleavage of an Enzyme Precursor 231
Some Regulatory Enzymes Use Several Regulatory Mechanisms 232
7 Carbohydrates and Glycobiology 238
7.1 Monosaccharides and Disaccharides 239
The Two Families of Monosaccharides Are Aldoses and Ketoses 239
Monosaccharides Have Asymmetric Centers 239
The Common Monosaccharides Have Cyclic Structures 240
Organisms Contain a Variety of Hexose Derivatives 243
Monosaccharides Are Reducing Agents 244
Disaccharides Contain a Glycosidic Bond 245
7.2 Polysaccharides 247
Some Homopolysaccharides Are Stored Forms of Fuel 247
Some Homopolysaccharides Serve Structural Roles 248
Steric Factors and Hydrogen Bonding Influence Homopolysaccharide Folding 250
Bacterial and Algal Cell Walls Contain Structural Heteropolysaccharides 252
Glycosaminoglycans Are Heteropolysaccharides of the Extracellular Matrix 253
7.3 Glycoconjugates: Proteoglycans, Glycoproteins, and Glycolipids 255
Proteoglycans Are Glycosaminoglycan-Containing Macromolecules of the Cell Surface and Extracellular Matrix 256
Glycoproteins Have Covalently Attached Oligosaccharides 258
Glycolipids and Lipopolysaccharides Are Membrane Components 260
7.4 Carbohydrates as Informational Molecules: The Sugar Code 261
Lectins Are Proteins That Read the Sugar Code and Mediate Many Biological Processes 262
Lectin-Carbohydrate Interactions Are Very Strong and Highly Specific 264
7.5 Working with Carbohydrates 267
8 Nucleotides and Nucleic Acids 273
8.1 Some Basics 273
Nucleotides and Nucleic Acids Have Characteristic Bases and Pentoses 273
Phosphodiester Bonds Link Successive Nucleotides in Nucleic Acids 276
The Properties of Nucleotide Bases Affect the Three-Dimensional Structure of Nucleic Acids 278
8.2 Nucleic Acid Structure 279
DNA Stores Genetic Information 280
DNA Molecules Have Distinctive Base Compositions 281
DNA Is a Double Helix 282
DNA Can Occur in Different Three-Dimensional Forms 283
Certain DNA Sequences Adopt Unusual Structures 285
Messenger RNAs Code for Polypeptide Chains 287
Many RNAs Have More Complex Three-Dimensional Structures 288
8.3 Nucleic Acid Chemistry 291
Double-Helical DNA and RNA Can Be Denatured 291
Nucleic Acids from Different Species Can Form Hybrids 292
Nucleotides and Nucleic Acids Undergo Nonenzymatic Transformations 293
Some Bases of DNA Are Methylated 296
The Sequences of Long DNA Strands Can Be Determined 296
The Chemical Synthesis of DNA Has Been Automated 298
8.4 Other Functions of Nucleotides 300
Nucleotides Carry Chemical Energy in Cells 300
Adenine Nucleotides Are Components of Many Enzyme Cofactors 301
Some Nucleotides Are Regulatory Molecules 302
9 DNA-Based Information Technologies 306
9.1 DNA Cloning: The Basics 306
Restriction Endonucleases and DNA Ligase Yield Recombinant DNA 307
Cloning Vectors Allow Amplification of Inserted DNA Segments 311
Specific DNA Sequences Are Detectable by Hybridization 314
Expression of Cloned Genes Produces Large Quantities of Protein 315
Alterations in Cloned Genes Produce Modified Proteins 316
9.2 From Genes to Genomes 317
DNA Libraries Provide Specialized Catalogs of Genetic Information 318
The Polymerase Chain Reaction Amplifies Specific DNA Sequences 319
Genome Sequences Provide the Ultimate Genetic Libraries 321
Box 9-1 A Potent Weapon In Forensic Medicine 322
9.3 From Genomes to Proteomes 325
Sequence or Structural Relationships Provide Information on Protein Function 325
Cellular Expression Patterns Can Reveal the Cellular Function of a Gene 326
Detection of Protein-Protein Interactions Helps to Define Cellular and Molecular Function 327
9.4 Genome Alterations and New Products of Biotechnology 330
A Bacterial Plant Parasite Aids Cloning in Plants 330
Manipulation of Animal Cell Genomes Provides Information on Chromosome Structure and Gene Expression 333
New Technologies Promise to Expedite the Discovery of New Pharmaceuticals 335
Box 9-2 The Human Genome and Human Gene Therapy 336
Recombinant DNA Technology Yields New Products and Challenges 338
10 Lipids 343
10.1 Storage Lipids 343
Fatty Acids Are Hydrocarbon Derivatives 343
Triacylglycerols Are Fatty Acid Esters of Glycerol 345
Triacylglycerols Provide Stored Energy and Insulation 346
Many Foods Contain Triacylglycerols 346
Box 10-1 Sperm Whales: Fatheads of the Deep 347
Waxes Serve as Energy Stores and Water Repellents 348
10.2 Structural Lipids in Membranes 348
Glycerophospholipids Are Derivatives of Phosphatidic Acid 349
Some Phospholipids Have Ether-Linked Fatty Acids 349
Chloroplasts Contain Galactolipids and Sulfolipids 351
Archaebacteria Contain Unique Membrane Lipids 352
Sphingolipids Are Derivatives of Sphingosine 352
Sphingolipids at Cell Surfaces Are Sites of Biological Recognition 353
Phospholipids and Sphingolipids Are Degraded in Lysosomes 354
Sterols Have Four Fused Carbon Rings 354
Box 10-2 Inherited Human Diseases Resulting from Abnormal Accumulations of Membrane Lipids 356
10.3 Lipids as Signals, Cofactors, and Pigments 357
Phosphatidylinositols and Sphingosine Derivatives Act as Intracellular Signals 357
Eicosanoids Carry Messages to Nearby Cells 358
Steroid Hormones Carry Messages between Tissues 359
Plants Use Phosphatidylinositols, Steroids, and Eicosanoidlike Compounds in Signaling 360
Vitamins A and D Are Hormone Precursors 360
Vitamins E and K and the Lipid Quinones Are Oxidation-Reduction Cofactors 362
Dolichols Activate Sugar Precursor for Biosynthesis 363
10.4 Working with Lipids 363
Lipid Extraction Requires Organic Solvents 364
Adsorption Chromatography Separates Lipids of Different Polarity 365
Gas-Liquid Chromatography Resolves Mixtures of Volatile Lipid Derivatives 365
Specific Hydrolysis Aids in Determination of Lipid Structure 365
Mass Spectrometry Reveals Complete Lipid Structure 365
11 Biological Membranes and Transport 369
11.1 The Composition and Architecture of Membranes 370
Each Type of Membrane Has Characteristic Lipids and Proteins 370
All Biological Membranes Share Some Fundamental Properties 371
A Lipid Bilayer Is the Basic Structural Element of Membranes 371
Peripheral Membrane Proteins Are Easily Solubilized 373
Many Membrane Proteins Span the Lipid Bilayer 373
Integral Proteins Are Held in the Membrane by Hydrophobic Interactions with Lipids 375
The Topology of an Integral Membrane Protein Can Be Predicted from Its Sequence 376
Covalently Attached Lipids Anchor Some Membrane Proteins 378
11.2 Membrane Dynamics 380
Acyl Groups in the Bilayer Interior Are Ordered to Varying Degrees 380
Transbilayer Movement of Lipids Requires Catalysis 381
Lipids and Proteins Diffuse Laterally in the Bilayer 382
Box 11-1 Atomic Force Microscopy to Visualize Membrane Proteins 384
Sphingolipids and Cholesterol Cluster Together in Membrane Rafts 383
Caveolins Define a Special Class of Membrane Rafts 385
Certain Integral Proteins Mediate Cell-Cell Interactions and Adhesion 385
Membrane Fusion Is Central to Many Biological Processes 387
11.3 Solute Transport across Membranes 389
Passive Transport Is Facilitated by Membrane Proteins 389
Transporters Can Be Grouped into Superfamilies Based onTheir Structures 391
The Glucose Transporter of Erythrocytes Mediates Passive Transport 393
The Chloride-Bicarbonate Exchanger Catalyzes Electroneutral Cotransport of Anions across the Plasma Membrane 395
Box 11-2 Defective Glucose and Water Transport In Two Forms of Diabetes 396
Active Transport Results in Solute Movement against a Concentration or Electrochemical Gradient 397
P-Type ATPases Undergo Phosphorylation during Their Catalytic Cycles 398
P-Type Ca 2+ Pumps Maintain a Low Concentration of Calcium in the Cytosol 400
F-Type ATPases Are Reversible, ATP-Driven Proton Pumps 401
ABC Transporters Use ATP to Drive the Active Transport of a Wide Variety of Substrates 402
Ion Gradients Provide the Energy for Secondary Active Transport 402
Box 11-3 A Defective Ion Channel in Cystic Fibrosis 403
Aquaporins Form Hydrophilic Transmembrane Channels for the Passage of Water 406
Ion-Selective Channels Allow Rapid Movement of Ions across Membranes 408
Ion-Channel Function Is Measured Electrically 408
The Structure of a K+ Channel Reveals the Basis for Its Specificity 409
The Neuronal Na+ Channel Is a Voltage-Gated Ion Channel 410
The Acetylcholine Receptor Is a Ligand-Gated Ion Channel 411
Defective Ion Channels Can Have Adverse Physiological Consequences 415
12 Biosignaling 421
12.1 Molecular Mechanisms of Signal Transduction 422
Box 12-1 Scatchard Analysis Quantifies the Receptor-Ligand Interaction 423
12.2 Gated Ion Channels 425
Ion Channels Underlie Electrical Signaling in Excitable Cells 425
The Nicotinic Acetylcholine Receptor Is a Ligand-Gated Ion Channel 426
Voltage-Gated Ion Channels Produce Neuronal Action Potentials 427
Neurons Have Receptor Channels That Respond to Different Neurotransmitters 428
12.3 Receptor Enzymes 429
The Insulin Receptor Is a Tyrosine-Specific Protein Kinase 429
Receptor Guanylyl Cyclases Generate the Second Messenger cGMP 433
12.4 G Protein-Coupled Receptors and Second Messengers 435
The β-Adrenergic Receptor System Acts through the Second Messenger cAMP 435
The β-Adrenergic Receptor Is Desensitized by Phosphotylation 439
Cyclic AMP Acts as a Second Messenger for a Number of Regulatory Molecules 441
Two Second Messengers Are Derived from Phosphatidylinositols 442
Calcium Is a Second Messenger in Many Signal Transductions 442
Box 12-2 FRET: Biochemistry Vlsuallzed in a Living Cell 446
12.5 Multivalent Scaffold Proteins and Membrane Rafts 448
Protein Modules Bind Phosphorylated Tyr, Ser, or Thr Residues in Partner Proteins 448
Membrane Rafts and Caveolae May Segregate Signaling Proteins 451
12.6 Signaling in Microorganisms and Plants 452
Bacterial Signaling Entails Phosphorylation in a Two-Component System 452
Signaling Systems of Plants Have Some of the Same Components Used by Microbes and Mammals 452
Plants Detect Ethylene through a Two-Component System and a MAPK Cascade 454
Receptorlike Protein Kinases Transduce Signals from Peptides and Brassinosteroids 455
12.7 Sensory Transduction in Vision, Olfaction, and Gustation 456
Light Hyperpolarizes Rod and Cone Cells of the Vertebrate Eye 456
Light Triggers Conformational Changes in the Receptor Rhodopsin 457
Excited Rhodopsin Acts through the G Protein Transducin to Reduce the cGMP Concentration 457
Amplification of the Visual Signal Occurs in the Rod and Cone Cells 458
The Visual Signal Is Quickly Terminated 458
Rhodopsin Is Desensitized by Phosphorylation 459
Cone Cells Specialize in Color Vision 460
Vertebrate Olfaction and Gustation Use Mechanisms Similar to the Visual System 460
Box 12-3 Color Blindness: John Dalton's Experiment from the Grave 461
G Protein-Coupled Serpentine Receptor Systems Share Several Features 462
Disruption of G-Protein Signaling Causes Disease 464
12.8 Regulation of Transcription by Steroid Hormones 465
12.9 Regulation of the Cell Cycle by Protein Kinases 466
The Cell Cycle Has Four Stages 466
Levels of Cyclin-Dependent Protein Kinases Oscillate 467
CDKs Regulate Cell Division by Phosphorylating Critical Proteins 470
12.10 Oncogenes, Tumor Suppressor Genes, and Programmed Cell Death 471
Oncogenes Are Mutant Forms of the Genes for Proteins That Regulate the Cell Cycle 471
Defects in Tumor Suppressor Genes Remove Normal Restraints on Cell Division 472
Apoptosis Is Programmed Cell Suicide 473
Ⅱ BIOENERGETICS AND METABOLISM 481
13 Principles of Bioenergetics 489
13.1 Bioenergetics and Thermodynamics 490
Biological Energy Transformations Obey the Laws of Thermodynamics 490
Cells Require Sources of Free Energy 491
The Standard Free-Energy Change Is Directly Related to the Equilibrium Constant 491
Actual Free-Energy Changes Depend on Reactant and Product Concentrations 493
Standard Free-Energy Changes Are Additive 494
13.2 Phosphoryl Group Transfers and ATP 496
The Free-Energy Change for ATP Hydrolysis Is Large and Negative 496
Other Phosphorylated Compounds and Thioesters Also Have Large Free Energies of Hydrolysis 497
Box 13-1 The Free Energy of Hydrolysis of ATP within Cells: The Real Cost of Doing Metabolic Business 498
ATP Provides Energy by Group Transfers, Not by Simple Hydrolysis 500
ATP Donates Phosphoryl, Pyrophosphoryl, and Adenylyl Groups 502
Box 13-2 Firefly Flashes: Glowing Reports of ATP 503
Assembly of Informational Macromolecules Requires Energy 504
ATP Energizes Active Transport and Muscle Contraction 504
Transphosphorylations between Nucleotides Occur in All Cell Types 505
Inorganic Polyphosphate Is a Potential Phosphoryl Group Donor 506
Biochemical and Chemical Equations Are Not Identical 506
13.3 Biological Oxidation-Reduction Reactions 507
The Flow of Electrons Can Do Biological Work 507
Oxidation-Reduction Can Be Described as Half-Reactions 508
Biological Oxidations Often Involve Dehydrogenation 508
Reduction Potentials Measure Affinity for Electrons 509
Standard Reduction Potentials Can Be Used to Calculate the Free-Energy Change 510
Cellular Oxidation of Glucose to Carbon Dioxide Requires Specialized Electron Carriers 512
A Few Types of Coenzymes and Proteins Serve as Universal Electron Carriers 512
NADH and NADPH Act with Dehydrogenases as Soluble Electron Carriers 512
Dietary Deficiency of Niacin, the Vitamin Form of NAD and NADP, Causes Pellagra 514
Flavin Nucleotides Are Tightly Bound in Flavoproteins 515
14 Glycolysis, Gluconeogenesis, and the Pentose Phosphate Pathway 521
14.1 Glycolysis 522
An Overview: Glycolysis Has Two Phases 523
The Preparatory Phase of Glycolysis Requires ATP 525
The Payoff Phase of Glycolysis Produces ATP and NADH 529
The Overall Balance Sheet Shows a Net Gain of ATP 533
Glycolysis Is under Tight Regulation 533
Cancerous Tissue Has Deranged Glucose Catabolism 533
14.2 Feeder Pathways for Glycolysis 534
Glycogen and Starch Are Degraded by Phosphorolysis 534
Dietary Polysaccharides and Disaccharides Undergo Hydrolysis to Monosaccharides 535
Other Monosaccharides Enter the Glycolytic Pathway at Several Points 536
14.3 Fates of Pyruvate under Anaerobic Conditions:Fermentation 538
Pyruvate Is the Terminal Electron Acceptor in Lactic Acid Fermentation 538
Ethanol Is the Reduced Product in Ethanol Fermentation 538
Box 14-1 Athletes, Alligators, and Coelacanths: Glycolysis at Limiting Concentrations of Oxygen 539
Thiamine Pyrophosphate Carries “Active Aldehyde” Groups 540
Fermentations Yield a Variety of Common Foods and Industrial Chemicals 541
Box 14-2 Brewing Beer 542
14.4 Gluconeogenesis 543
Conversion of Pyruvate to Phosphoenolpyruvate Requires Two Exergonic Reactions 544
Conversion of Fructose 1,6-Bisphosphate to Fructose 6-Phosphate Is the Second Bypass 547
Conversion of Glucose 6-Phosphate to Glucose Is the Third Bypass 547
Gluconeogenesis Is Energetically Expensive, But Essential 548
Citric Acid Cycle Intermediates and Many Amino Acids Are Glucogenic 548
Glycolysis and Gluconeogenesis Are Regulated Reciprocally 548
14.5 Pentose Phosphate Pathway of Glucose Oxidation 549
The Oxidative Phase Produces Pentose Phosphates and NADPH 550
Box 14-3 Why Pythagoras Wouldn't Eat Falafel: Glucose 6-Phosphate Dehydrogenase Deficiency 551
The Nonoxidative Phase Recycles Pentose Phosphates to Glucose 6-Phosphate 552
Wernicke-Korsakoff Syndrome Is Exacerbated by a Defect in Transketolase 554
Glucose 6-Phosphate Is Partitioned between Glycolysis and the Pentose Phosphate Pathway 554
15 Principles of Metabolic Regulation:Glucose and Glycogen 560
15.1 The Metabolism of Glycogen in Animals 562
Glycogen Breakdown Is Catalyzed by Glycogen Phosphorylase 562
Glucose 1-Phosphate Can Enter Glycolysis or, in Liver,Replenish Blood Glucose 563
The Sugar Nucleotide UDP-Glucose Donates Glucose for Glycogen Synthesis 565
Box 15-1 Carl and Gerty Cori: Pioneers in Glycogen Metabollsm and Disease 566
Glycogenin Primes the Initial Sugar Residues in Glycogen 569
15.2 Regulation of Metabolic Pathways 571
Living Cells Maintain a Dynamic Steady State 571
Regulatory Mechanisms Evolved under Strong Selective Pressures 571
Regulatory Enzymes Respond to Changes in Metabolite Concentration 572
Enzyme Activity Can Be Altered in Several Ways 574
15.3 Coordinated Regulation of Glycolysis and Gluconeogenesis 575
Hexokinase Isozymes of Muscle and Liver Are Affected Differently by Their Product, Glucose 6-Phosphate 576
Box 15-2 Isozymes: Different Proteins That Catalyze the Same Reaction 577
Phosphofructokinase-1 Is under Complex Allosteric Regulation 578
Pyruvate Kinase Is Allosterically Inhibited by ATP 579
Gluconeogenesis Is Regulated at Several Steps 580
Fructose 2,6-Bisphosphate Is a Potent Regulator of Glycolysis and Gluconeogenesis 581
Are Substrate Cycles Futile? 583
Xylulose 5-Phosphate Is a Key Regulator of Carbohydrate and Fat Metabolism 583
15.4 Coordinated Regulation of Glycogen Synthesis and Breakdown 583
Glycogen Phosphorylase Is Regulated Allosterically and Hormonally 583
Glycogen Synthase Is Also Regulated by Phosphorylation and Dephosphorylation 586
Glycogen Synthase Kinase 3 Mediates the Actions of Insulin 586
Phosphoprotein Phosphatase 1 Is Central to Glycogen Metabolism 588
Transport into Cells Can Limit Glucose Utilization 588
Allosteric and Hormonal Signals Coordinate Carbohydrate Metabolism 588
Carbohydrate and Lipid Metabolism Are Integrated by Hormonal and Allosteric Mechanisms 590
Insulin Changes the Expression of Many Genes Involved in Carbohydrate and Fat Metabolism 590
15.5 Analysis of Metabolic Control 591
The Contribution of Each Enzyme to Flux through a Pathway Is Experimentally Measurable 592
The Control Coefficient Quantifies the Effect of a Change in Enzyme Activity on Metabolite Flux through a Pathway 592
The Elasticity Coefficient Is Related to an Enzyme's Responsiveness to Changes in Metabolite or Regulator Concentrations 593
The Response Coefficient Expresses the Effect of an Outside Controller on Flux through a Pathway 593
Metabolic Control Analysis Has Been Applied to Carbohydrate Metabolism, with Surprising Results 593
Box 15-3 Metabolic Control Analysis: Quantitative Aspects 594
Metabolic Control Analysis Suggests a General Method for Increasing Flux through a Pathway 596
16 The Citric Acid Cycle 601
16.1 Production of Acetyl-CoA (Activated Acetate) 602
Pyruvate Is Oxidized to Acetyl-CoA and CO2 602
The Pyruvate Dehydrogenase Complex Requires Five Coenzymes 603
The Pyruvate Dehydrogenase Complex Consists of Three Distinct Enzymes 604
In Substrate Channeling, Intermediates Never Leave the Enzyme Surface 605
16.2 Reactions of the Citric Acld Cycle 606
The Citric Acid Cycle Has Eight Steps 608
Box 16-1 Synthases and Synthetases; Llgases and Lyases; Klnases,Phosphatases, and Phosphorylases: Yes, the Names Are Confusing! 613
The Energy of Oxidations in the Cycle Is Efficiently Conserved 614
Box 16-2 Cltrate: A Symmetrlcal Molecule That Reacts Asymmetrlcally 614
Why Is the Oxidation of Acetate So Complicated? 615
Citric Acid Cycle Components Are Important Biosynthetic Intermediates 616
Anaplerotic Reactions Replenish Citric Acid Cycle Intermediates 616
Box 16-3 Citrate Synthase, Soda Pop, and the World Food Supply 618
Biotin in Pyruvate Carboxylase Carries CO2 Groups 618
16.3 Regulation of the Citric Acid Cycle 621
Production of Acetyl-CoA by the Pyruvate Dehydrogenase Complex Is Regulated by Allosteric and Covalent Mechanisms 621
The Citric Acid Cycle Is Regulated at Its Three Exergonic Steps 622
Substrate Channeling through Multienzyme Complexes May Occur in the Citric Acid Cycle 622
16.4 The Glyoxylate Cycle 623
The Glyoxylate Cycle Produces Four-Carbon Compounds from Acetate 623
The Citric Acid and Glyoxylate Cycles Are Coordinately Regulated 624
17 Fatty Acid Catabolism 631
17.1 Digestion, Mobilization, and Transport of Fats 632
Dietary Fats Are Absorbed in the Small Intestine 632
Hormones Trigger Mobilization of Stored Triacylglycerols 634
Fatty Acids Are Activated and Transported into Mitochondria 634
17.2 Oxidation of Fatty Acids 637
The β Oxidation of Saturated Fatty Acids Has Four Basic Steps 637
The Four β-Oxidation Steps Are Repeated to Yield Acetyl-CoA and ATP 639
Acetyl-CoA Can Be Further Oxidized in the Citric Acid Cycle 639
Oxidation of Unsaturated Fatty Acids Requires Two Additional Reactions 639
Box 17-1 Fat Bears Carry Out β Oxldatlon In Thelr Sleep 640
Complete Oxidation of Odd-Number Fatty Acids Requires Three Extra Reactions 642
Fatty Acid Oxidation Is Tightly Regulated 642
Genetic Defects in Fatty Acyl-CoA Dehydrogenases Cause Serious Disease 643
Box 17-2 Coenzyme B12: A Radlcal Solutlon to a Perplexing Problem 644
Peroxisomes Also Carry Out β Oxidation 646
Plant Peroxisomes and Glyoxysomes Use Acetyl-CoA from β Oxidation as a Biosynthetic Precursor 647
The β-Oxidation Enzymes of Different Organelles Have Diverged during Evolution 647
The ω Oxidation of Fatty Acids Occurs in the Endoplasmic Reticulum 647
Phytanic Acid Undergoes α Oxidation in Peroxisomes 649
17.3 Ketone Bodies 650
Ketone Bodies, Formed in the Liver, Are Exported to Other Organs as Fuel 650
Ketone Bodies Are Overproduced in Diabetes and during Starvation 652
18 Amino Acid Oxidation and the Production of Urea 656
18.1 Metabolic Fates of Amino Groups 657
Dietary Protein Is Enzymatically Degraded to Amino Acids 658
Pyridoxal Phosphate Participates in the Transfer of α-Amino Groups to α-Ketoglutarate 660
Glutamate Releases its Amino Group as Ammonia in the Liver 661
Glutamine Transports Ammonia in the Bloodstream 662
Box 18-1 Assays for Tissue Damage 664
Alanine Transports Ammonia from Skeletal Muscles to the Liver 664
Ammonia Is Toxic to Animals 665
18.2 Nitrogen Excretion and the Urea Cycle 665
Urea Is Produced from Ammonia in Five Enzymatic Steps 667
The Citric Acid and Urea Cycles Can Be Linked 668
The Activity of the Urea Cycle Is Regulated at Two Levels 669
Pathway Interconnections Reduce the Energetic Cost of Urea Synthesis 669
Genetic Defects in the Urea Cycle Can Be Life-Threatening 669
18.3 Pathways of Amino Acid Degradation 671
Some Amino Acids Are Converted to Glucose, Others to Ketone Bodies 671
Several Enzyme Cofactors Play Important Roles in Amino Acid Catabolism 672
Six Amino Acids Are Degraded to Pyruvate 674
Seven Amino Acids Are Degraded to Acetyl-CoA 677
Phenylalanine Catabolism Is Genetically Defective in Some People 679
Five Amino Acids Are Converted to α-Ketoglutarate 681
Four Amino Acids Are Converted to Succinyl-CoA 682
Branched-Chain Amino Acids Are Not Degraded in the Liver 683
Box 18-2 Sclentlflc Sleuths Solve a Murder Mystery 684
Asparagine and Aspartate Are Degraded to Oxaloacetate 685
19 Oxidative Phosphorylation and Photophosphorylation 690
OXIDATIVE PHOSPHORYLATION 691
19.1 Electron-Transfer Reactions in Mitochondria 691
Electrons Are Funneled to Universal Electron Acceptors 692
Electrons Pass through a Series of Membrane-Bound Carriers 693
Electron Carriers Function in Multienzyme Complexes 696
The Energy of Electron Transfer Is Efficiently Conserved in a Proton Gradient 701
Plant Mitochondria Have Alternative Mechanisms for Oxidizing NADH 704
19.2 ATP Synthesis 704
Box 19-1 Hot, Stlnklng Plants and Alternatlve Resplratory Pathways 706
ATP Synthase Has Two Functional Domains,Fo and F1 708
ATP Is Stabilized Relative to ADP on the Surface of F1 708
The Proton Gradient Drives the Release of ATP from the Enzyme Surface 709
Each β Subunit of ATP Synthase Can Assume Three Different Conformations 709
Rotational Catalysis Is Key to the Binding-Change Mechanism for ATP Synthesis 711
Chemiosmotic Coupling Allows Nonintegral Stoichiometries of O2 Consumption and ATP Synthesis 712
The Proton-Motive Force Energizes Active Transport 713
Shuttle Systems Indirectly Convey Cytosolic NADH into Mitochondria for Oxidation 714
19.3 Regulation of Oxidative Phosphorylation 716
Oxidative Phosphorylation Is Regulated by Cellular Energy Needs 716
An Inhibitory Protein Prevents ATP Hydrolysis during Ischemia 717
Uncoupled Mitochondria in Brown Fat Produce Heat 717
ATP-Producing Pathways Are Coordinately Regulated 718
19.4 Mitochondrial Genes: Their Origin and the Effects of Mutations 719
Mutations in Mitochondrial Genes Cause Human Disease 719
Mitochondria Evolved from Endosymbiotic Bacteria 72119.5 The Role of Mitochondria in Apoptosis and OxidativeStress 721PHOTOSYNTHESIS: HARVESTING LIGHT ENERGY 72319.6 General Features of Photophosphorylation 723
Photosynthesis in Plants Takes Place in Chloroplasts 724
Light Drives Electron Flow in Chloroplasts 724
19.7 Light Absorption 725
Chlorophylls Absorb Light Energy for Photosynthesis 725
Accessory Pigments Extend the Range of Light Absorption 728
Chlorophyll Funnels the Absorbed Energy to Reaction Centers by Exciton Transfer 728
19.8 The Central Photochemical Event: Light-Driven Electron Flow 730
Bacteria Have One of Two Types of Single Photochemical Reaction Center 730
Kinetic and Thermodynamic Factors Prevent the Dissipation of Energy by Internal Conversion 732
In Plants, Two Reaction Centers Act in Tandem 733
Antenna Chlorophylls Are Tightly Integrated with Electron Carriers 734
Spatial Separation of Photosystems Ⅰ and Ⅱ Prevents Exciton Larceny 736
The Cytochrome b6 f Complex Links Photosystems Ⅱ and I 737
Cyanobacteria Use the Cytochrome b6 f Complex and Cytochrome c6 in Both Oxidative Phosphorylation and Photophosphorylation 738
Water Is Split by the Oxygen-Evolving Complex 738
19.9 ATP Synthesis by Photophosphorylation 740
A Proton Gradient Couples Electron Flow and Photophosphorylation 740
The Approximate Stoichiometry of Photophosphorylation Has Been Established 741
Cyclic Electron Flow Produces ATP but Not NADPH or O2 741
The ATP Synthase of Chloroplasts Is Like That of Mitochondria 742
Chloroplasts Evolved from Endosymbiotic Bacteria 742
Diverse Photosynthetic Organisms Use Hydrogen Donors Other Than Water 743
In Halophilic Bacteria, a Single Protein Absorbs Light and Pumps Protons to Drive ATP Synthesis 743
20 Carbohydrate Biosynthesis in Plants and Bacteria 751
20.1 Photosynthetic Carbohydrate Synthesis 751
Plastids Are Organelles Unique to Plant Cells and Algae 752
Carbon Dioxide Assimilation Occurs in Three Stages 753
Synthesis of Each Triose Phosphate from CO2 Requires Six NADPH and Nine ATP 762
A Transport System Exports Triose Phosphates from the Chloroplast and Imports Phosphate 763
Four Enzymes of the Calvin Cycle Are Indirectly Activated by Light 764
20.2 Photorespiration and the C4 and CAM Pathways 766
Photorespiration Results from Rubisco's Oxygenase Activity 766
The Salvage of Phosphoglycolate Is Costly 767
In C4 Plants, CO2 Fixation and Rubisco Activity Are Spatially Separated 769
In CAM Plants, CO2 Capture and Rubisco Action Are Temporally Separated 770
20.3 Biosynthesis of Starch and Sucrose 771
ADP-Glucose Is the Substrate for Starch Synthesis in Plant Plastids and for Glycogen Synthesis in Bacteria 771
UDP-Glucose Is the Substrate for Sucrose Synthesis in the Cytosol of Leaf Cells 771
Conversion of Triose Phosphates to Sucrose and Starch Is Tightly Regulated 772
20.4 Synthesis of Cell Wall Polysaccharides: Plant Cellulose and Bacterial Peptidoglycan 775
Cellulose Is Synthesized by Supramolecular Structures in the Plasma Membrane 775
Lipid-Linked Oligosaccharides Are Precursors for Bacterial Cell Wall Synthesis 777
Box 20-1 The Magic Bullet versus the Bulletproof Vest: Penicillin and β-Lactamase 779
20.5 Integration of Carbohydrate Metabolism in the Plant Cell 780
Gluconeogenesis Converts Fats and Proteins to Glucose in Germinating Seeds 780
Pools of Common Intermediates Link Pathways in Different Organelles 781
21 Lipid Biosynthesis 787
21.1 Biosynthesis of Fatty Acids and Eicosanoids 787
Malonyl-CoA Is Formed from Acetyl-CoA and Bicarbonate 787
Fatty Acid Synthesis Proceeds in a Repeating Reaction Sequence 788
The Fatty Acid Synthase Complex Has Seven Different Active Sites 789
Fatty Acid Synthase Receives the Acetyl and Malonyl Groups 790
The Fatty Acid Synthase Reactions Are Repeated to Form Palmitate 791
The Fatty Acid Synthase of Some Organisms Consists of Multifunctional Proteins 794
Fatty Acid Synthesis Occurs in the Cytosol of Many Organisms but in the Chloroplasts of Plants 794
Acetate Is Shuttled out of Mitochondria as Citrate 794
Fatty Acid Biosynthesis Is Tightly Regulated 795
Long-Chain Saturated Fatty Acids Are Synthesized from Palmitate 797
Desaturation of Fatty Acids Requires a Mixed-Function Oxidase 798
Box 21-1 Mixed-Function Oxidases, Oxygenases, and Cytochrome P-450 798
Eicosanoids Are Formed from 20-Carbon Polyunsaturated Fatty Acids 800
Box 21-2 Relief Is in (the Active) Site: Cyclooxygenase Isozymes and the Search for a Better Aspirin 802
21.2 Biosynthesis of Triacylglycerols 804
Triacylglycerols and Glycerophospholipids Are Synthesized from the Same Precursors 804
Triacylglycerol Biosynthesis in Animals Is Regulated by Hormones 804
Adipose Tissue Generates Glycerol 3-phosphate by Glyceroneogenesis 806
21.3 Biosynthesis of Membrane Phospholipids 808
Cells Have Two Strategies for Attaching Phospholipid Head Groups 809
Phospholipid Synthesis in E.coli Employs CDP-Diacylglycerol 811
Eukaryotes Synthesize Anionic Phospholipids from CDP-Diacylglycerol 811
Eukaryotic Pathways to Phosphatidylserine,Phosphatidylethanolamine, and Phosphatidylcholine Are Interrelated 812
Plasmalogen Synthesis Requires Formation of an Ether-Linked Fatty Alcohol 813
Sphingolipid and Glycerophospholipid Synthesis Share Precursors and Some Mechanisms 813
Polar Lipids Are Targeted to Specific Cellular Membranes 814
21.4 Biosynthesis of Cholesterol, Steroids, and Isoprenoids 816
Cholesterol Is Made from Acetyl-CoA in Four Stages 816
Cholesterol Has Several Fates 820
Cholesterol and Other Lipids Are Carried on Plasma Lipoproteins 820
Box 21-3 ApoE Alleles Predict Incidence of Alzheimer's Disease 824
Cholesteryl Esters Enter Cells by Receptor-Mediated Endocytosis 824
Cholesterol Biosynthesis Is Regulated at Several Levels 825
Steroid Hormones Are Formed by Side-Chain Cleavage and Oxidation of Cholesterol 827
Intermediates in Cholesterol Biosynthesis Have Many Alternative Fates 828
22 Biosynthesis of Amino Acids, Nucleotides,and Related Molecules 833
22.1 Overview of Nitrogen Metabolism 834
The Nitrogen Cycle Maintains a Pool of Biologically Available Nitrogen 834
Nitrogen Is Fixed by Enzymes of the Nitrogenase Complex 834
Ammonia Is Incorporated into Biomolecules through Glutamate and Glutamine 837
Glutamine Synthetase Is a Primary Regulatory Point in Nitrogen Metabolism 838
Several Classes of Reactions Play Special Roles in the Biosynthesis of Amino Acids and Nucleotides 840
22.2 Biosynthesis of Amino Acids 841
α-Ketoglutarate Gives Rise to Glutamate, Glutamine, Proline,and Arginine 842
Serine, Glycine, and Cysteine Are Derived from 3-Phospho-glycerate 842
Three Nonessential and Six Essential Amino Acids Are Synthesized from Oxaloacetate and Pyruvate 845
Chorismate Is a Key Intermediate in the Synthesis of Tryptophan, Phenylalanine, and Tyrosine 849
Histidine Biosynthesis Uses Precursors of Purine Biosynthesis 851
Amino Acid Biosynthesis Is under Allosteric Regulation 851
22.3 Molecules Derived from Amino Acids 854
Glycine Is a Precursor of Porphyrins 854
Heme Is the Source of Bile Pigments 854
Box 22-1 Blochemistry of Kings and Vampires 857
Amino Acids Are Precursors of Creatine and Glutathione 857
D-Amino Acids Are Found Primarily in Bacteria 858
Aromatic Amino Acids Are Precursors of Many Plant Substances 859
Biological Amines Are Products of Amino Acid Decarboxylation 859
Arginine Is the Precursor for Biological Synthesis of Nitric Oxide 860
Box 22-2 Curing African Sleeping Sickness wlth a Biochemical Trojan Horse 862
22.4 Biosynthesis and Degradation of Nucleotides 862
De Novo Purine Nucleotide Synthesis Begins with PRPP 864
Purine Nucleotide Biosynthesis Is Regulated by Feedback Inhibition 866
Pyrimidine Nucleotides Are Made from Aspartate, PRPP, and Carbamoyl Phosphate 867
Pyrimidine Nucleotide Biosynthesis Is Regulated by Feedback Inhibition 868
Nucleoside Monophosphates Are Converted to Nucleoside Triphosphates 868
Ribonucleotides Are the Precursors of Deoxyribonucleotides 869
Thymidylate Is Derived from dCDP and dUMP 872
Degradation of Purines and Pyrimidines Produces Uric Acid and Urea, Respectively 873
Purine and Pyrimidine Bases Are Recycled by Salvage Pathways 875
Excess Uric Acid Causes Gout 875
Many Chemotherapeutic Agents Target Enzymes in the Nucleotide Biosynthetic Pathways 876
23 Hormonal Regulation and Integration of Mammalian Metabolism 881
23.1 Hormones: Diverse Structures for Diverse Functions 881
The Discovery and Purification of Hormones Require a Bioassay 882
Box 23-1 How Is a Hormone Dlscovered? The Arduous Path to Purlfied Insulin 883
Hormones Act through Specific High-Affinity Cellular Receptors 884
Hormones Are Chemically Diverse 886
Hormone Release Is Regulated by a Hierarchy of Neuronal and Hormonal Signals 889
23.2 Tissue-Specific Metabolism: The Dlvision of Labor 892
The Liver Processes and Distributes Nutrients 893
Adipose Tissue Stores and Supplies Fatty Acids 897
Muscles Use ATP for Mechanical Work 898
The Brain Uses Energy for Transmission of Electrical Impulses 900
Blood Carries Oxygen, Metabolites, and Hormones 900
23.3 Hormonal Regulatlon of Fuel Metabollsm 902
The Pancreas Secretes Insulin or Glucagonin Response to Changes in Blood Glucose 902
Insulin Counters High Blood Glucose 904
Glucagon Counters Low Blood Glucose 904
During Fasting and Starvation, Metabolism Shifts to Provide Fuel for the Brain 906
Epinephrine Signals Impending Activity 908
Cortisol Signals Stress, Including Low Blood Glucose 909
Diabetes Mellitus Arises from Defects in Insulin Production or Action 909
23.4 Obesity and the Regulation of Body Mass 910
The Lipostat Theory Predicts the Feedback Regulation of Adipose Tissue 910
Leptin Stimulates Production of Anorexigenic Peptide Hormones 912
Leptin Triggers a Signaling Cascade That Regulates Gene Expression 913
The Leptin System May Have Evolved to Regulate the Starvation Response 913
Insulin Acts in the Arcuate Nucleus to Regulate Eating and Energy Conservation 914
Adiponectin Acts through AMPK 914
Diet Regulates the Expression of Genes Central to Maintaining Body Mass 915
Short-Term Eating Behavior Is Set by Ghrelin and PYY3-36 916
Ⅲ INFORMATION PATHWAYS 921
24 Genes and Chromosomes 923
24.1 Chromosomal Elements 924
Genes Are Segments of DNA That Code for Polypeptide Chains and RNAs 924
DNA Molecules Are Much Longer Than the Cellular Packages That Contain Them 925
Eukaryotic Genes and Chromosomes Are Very Complex 928
24.2 DNA Supercoiling 930
Most Cellular DNA Is Underwound 932
DNA Underwinding Is Defined by Topological Linking Number 933
Topoisomerases Catalyze Changes in the Linking Number of DNA 935
DNA Compaction Requires a Special Form of Supercoiling 937
24.3 The Structure of Chromosomes 938
Chromatin Consists of DNA and Proteins 938
Histones Are Small, Basic Proteins 939
Nucleosomes Are the Fundamental Organizational Units of Chromatin 940
Nucleosomes Are Packed into Successively Higher Order Structures 942
Condensed Chromosome Structures Are Maintained by SMC Proteins 943
Bacterial DNA Is Also Highly Organized 943
25 DNA Metabolism 948
25.1 DNA Replication 950
DNA Replication Follows a Set of Fundamental Rules 950
DNA Is Degraded by Nucleases 952
DNA Is Synthesized by DNA Polymerases 952
Replication Is Very Accurate 954
E.coli Has at Least Five DNA Polymerases 955
DNA Replication Requires Many Enzymes and Protein Factors 957
Replication of the E.coli Chromosome Proceeds in Stages 958
Bacterial Replication Is Organized in Membrane-Bound Replication Factories 963
Replication in Eukaryotic Cells Is More Complex 964
25.2 DNA Repair 966
Mutations Are Linked to Cancer 966
All Cells Have Multiple DNA Repair Systems 967
Box 25-1 DNA Repair and Cancer 970
The Interaction of Replication Forks with DNA Damage Can Lead to Error-Prone Translesion DNA Synthesis 976
25.3 DNA Recombination 978
Homologous Genetic Recombination Has Several Functions 979
Recombination during Meiosis Is Initiated with Double-Strand Breaks 980
Recombination Requires a Host of Enzymes and Other Proteins 982
All Aspects of DNA Metabolism Come Together to Repair Stalled Replication Forks 984
Site-Specific Recombination Results in Precise DNA Rearrangements 984
Complete Chromosome Replication Can Require Site-Specific Recombination 988
Transposable Genetic Elements Move from One Location to Another 988
Immunoglobulin Genes Assemble by Recombination 990
26 RNA Metabolism 995
26.1 DNA-Dependent Synthesis of RNA 996
RNA Is Synthesized by RNA Polymerases 996
RNA Synthesis Begins at Promoters 998
Transcription Is Regulated at Several Levels 1001
Specific Sequences Signal Termination of RNA Synthesis 1001
Box 26-1 RNA Polymerase Leaves Its Footprint on a Promoter 1002
Eukaryotic Cells Have Three Kinds of Nuclear RNA Polymerases 1003
RNA Polymerase Ⅱ Requires Many Other Protein Factors for Its Activity 1003
DNA-Dependent RNA Polymerase Undergoes Selective Inhibition 1006
26.2 RNA Processing 1007
Eukaryotic mRNAs Are Capped at the 5' End 1008
Both Introns and Exons Are Transcribed from DNA into RNA 1008
RNA Catalyzes the Splicing of Introns 1009
Eukaryotic mRNAs Have a Distinctive 3' End Structure 1011
A Gene Can Give Rise to Multiple Products by Differential RNA Processing 1014
Ribosomal RNAs and tRNAs Also Undergo Processing 1014
RNA Enzymes Are the Catalysts of Some Events in RNA Metabolism 1017
Cellular mRNAs Are Degraded at Different Rates 1020
Polynucleotide Phosphorylase Makes Random RNA-like Polymers 1020
26.3 RNA-Dependent Synthesis of RNA and DNA 1021
Reverse Transcriptase Produces DNA from Viral RNA 1021
Some Retroviruses Cause Cancer and AIDS 1023
Many Transposons, Retroviruses, and Introns May Have a Common Evolutionary Origin 1023
Box 26-2 Fighting AIDS with Inhibitors of HIV Reverse Transcriptase 1024
Telomerase Is a Specialized Reverse Transcriptase 1025
Some Viral RNAs Are Replicated by RNA-Dependent RNA Polymerase 1027
RNA Synthesis Offers Important Clues to Biochemical Evolution 1027
Box 26-3 The SELEX Method for Generating RNA Polymers with New Functlons 1030
27 Protein Metabolism 1034
27.1 The Genetic Code 1034
The Genetic Code Was Cracked Using Artificial mRNA Templates 1035
Wobble Allows Some tRNAs to Recognize More than One Codon 1039
Box 27-1 Changing Horses in Midstream: Translational Frameshiftlng and mRNA Editing 1040
Box 27-2 Exceptions That Prove the Rule: Natural Variations In the Genetic Code 1042
27.2 Protein Synthesis 1044
Protein Biosynthesis Takes Place in Five Stages 1044
The Ribosome Is a Complex Supramolecular Machine 1045
Box 27-3 From an RNA World to a Protein World 1048
Transfer RNAs Have Characteristic Structural Features 1049
Stage 1: Aminoacyl-tRNA Synthetases Attach the Correct Amino Acids to Their tRNAs 1051
Stage 2: A Specific Amino Acid Initiates Protein Synthesis 1054
Stage 3: Peptide Bonds Are Formed in the Elongation Stage 1058
Stage 4: Termination of Polypeptide Synthesis Requires a Special Signal 1061
Stage 5: Newly Synthesized Polypeptide Chains Undergo Folding and Processing 1062
Box 27-4 Induced Varlatlon in the Genetlc Code: Nonsense Suppresslon 1065
Protein Synthesis Is Inhibited by Many Antibiotics and Toxins 1065
27.3 Protein Targeting and Degradation 1068
Post translational Modification of Many Eukaryotic Proteins Begins in the Endoplasmic Reticulum 1068
Glycosylation Plays a Key Role in Protein Targeting 1069
Signal Sequences for Nuclear Transport Are Not Cleaved 1071
Bacteria Also Use Signal Sequences for Protein Targeting 1072
Cells Import Proteins by Receptor-Mediated Endocytosis 1074
Protein Degradation Is Mediated by Specialized Systems in All Cells 1075
28 Regulation of Gene Expression 1081
28.1 Principles of Gene Regulation 1082
RNA Polymerase Binds to DNA at Promoters 1082
Transcription Initiation Is Regulated by Proteins That Bind to or Near Promoters 1083
Many Prokaryotic Genes Are Clustered and Regulated in Operons 1085
The lac Operon Is Subject to Negative Regulation 1085
Regulatory Proteins Have Discrete DNA-Binding Domains 1087
Regulatory Proteins Also Have Protein-Protein Interaction Domains 1090
28.2 Regulation of Gene Expression in Prokaryotes 1092
The lac Operon Undergoes Positive Regulation 1093
Many Genes for Amino Acid Biosynthetic Enzymes Are Regulated by Transcription Attenuation 1094
Induction of the SOS Response Requires Destruction of Repressor Proteins 1097
Synthesis of Ribosomal Proteins Is Coordinated with rRNA Synthesis 1098
Some Genes Are Regulated by Genetic Recombination 1100
28.3 Regulation of Gene Expression in Eukaryotes 1102
Transcriptionally Active Chromatin Is Structurally Distinct from Inactive Chromatin 1102
Chromatin Is Remodeled by Acetylation and Nucleosomal Displacements 1103
Many Eukaryotic Promoters Are Positively Regulated 1103
DNA-Binding Transactivators and Coactivators Facilitate Assembly of the General Transcription Factors 1104
The Genes of Galactose Metabolism in Yeast Are Subject to Both Positive and Negative Regulation 1106
DNA-Binding Transactivators Have a Modular Structure 1106
Eukaryotic Gene Expression Can Be Regulated by Intercellular and Intracellular Signals 1108
Regulation Can Result from Phosphorylation of Nuclear Transcription Factors 1109
Many Eukaryotic mRNAs Are Subject to Translational Repression 1109
Posttranscriptional Gene Silencing Is Mediated by RNA Interference 1110
Development Is Controlled by Cascades of Regulatory Proteins 1111
Appendix A Common Abbreviations in the Biochemical Research Literature A-1 1148
Appendix B Abbreviated Solutions to Problems AS-1 1152
Glossary G-1 1121
Credits C-1 1139
Index I-1 1174