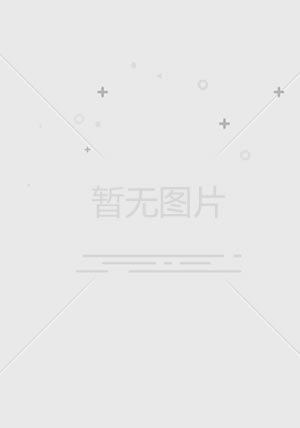
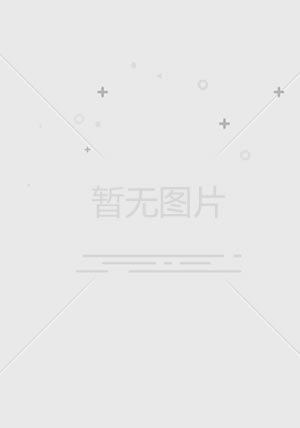
introduction to plant physiologyPDF电子书下载
- 电子书积分:20 积分如何计算积分?
- 作 者:
- 出 版 社:
- 出版年份:2222
- ISBN:
- 页数:0 页
Chapter 1 · Cells, Tissues, and Organs:The Architecture of Plants 1
1.1 The Plant Cell 2
1.2 Building Blocks: Lipids, Proteins,and Carbohydrates 2
1.2.1 Lipids Are a Class of Molecules That Includes Fats,Oils, Sterols, and Pigments 4
1.2.2 Proteins Play a Central Role in the Biochemistry of Cells and Are Responsible for Virtually All the Properties of Life as We Know it 6
1.2.3 Carbohydrates Are the Most Abundant Class of Biological Molecules 8
1.3 Biological Membranes 11
1.3.1 The Membrane Lipid Forms a Bilayer, a Highly Fluid but Very Stable Structure 11
1.3.2 Membranes Contain Significant Amounts of Protein 12
1.4 Cellular Organelles 13
1.4.1 Most Mature Plant Cells Contain a Large, Central Vacuole 13
1.4.2 The Nucleus Is the Information Center of the Cell 13
1.4.3 The Endoplasmic Reticulum and Golgi Apparatus Are Centers of Membrane Biosynthesis and Secretory Activities 14
1.4.4 The Mitochondrion Is the Principal Site of Cellular Respiration 15
1.4.5 Plastids Are a Family of Organelles with a Variety of Functions 15
1.4.6 Microbodies Are Metabolically Very Active 16
1.5 Cytoskeleton 16
1.6 The Extracellular Matrix 17
1.6.1 The Primary Cell Wall Is a Flexible Network of Cellulose Microfibrils and Cross-Linking Glycans 17
1.6.2 The Cellulose-Glycan Lattice is Embedded in a Matrix of Pectin and Protein 19
1.6.3 Cellulose Microfibrils Are Assembled at the Plasma Membrane as They Are Extruded into the Cell Wall 20
1.6.4 The Secondary Cell Wall Is Deposited on the Inside of the Primary Wall in Maturing Cells 21
1.6.5 Plasmadesmata Are Cytoplasmic Channels Extend Through the Wall to Connect the Protoplasts of Adjacent Cells 21
1.7 Tissues and Organs 22
1.7.1 Tissues Are Groups of Cells That Form an Organized, Functional Unit 22
1.7.2 Meristems Are Regions of Perpetually Dividing Cells 22
1.7.3 Parenchyma Is the Most Abundant Living Tissue in Plants 24
1.7.4 Supporting Tissues Are Distributed Throughout the Primary and Secondary Plant Bodies 24
1.7.5 Vascular Tissues Are the Principal Conducting Tissues for Water and Nutrients 25
1.7.6 Epidermis Is a Superficial Tissue That Forms a Continuous Layer over the Surface of the Primary Plant Body 25
1.8 Plant Organs 26
1.8.1 Roots Anchor the Plant and Absorb Water and Minerals from the Soil 26
1.8.2 Stems Elevate the Photosynthetic Organs, the Leaves, Toward the Sun 26
1.8.3 Leaves Are the Principal Photosynethic Organs 27
Summary 27
Chapter Review 28
Further Reading 28
Part 1 · Plants and Energy 29
Chapter 2 · Bioenergetics and ATP Synthesis 31
2.1 Bioenergetics and Energy Transformations in Living Organisms 31
2.1.1 The Sun Is a Primary Source of Energy 31
2.1.2 What Is Bioenergetics? 32
2.1.3 The First Law of Thermodynamics Refers to Energy Conservation 32
2.1.4 The Second Law of Thermodynamics Refers to Entropy and Disorder 33
2.1.5 The Ability to Do Work is Dependent on the Availability of Free Energy 33
2.1.6 Free Energy Is Related to Chemical Equilibria 34
2.2 Energy Transformations and Coupled Reactions 35
2.2.1 Free Energy of ATP Is Associated with Coupled Phosphate Transfer Reactions 35
2.2.2 Free Energy Changes Are Associated with Coupled Oxidation-Reduction Reactions 36
2.3 Energy Transduction and the Chemiosmotic Synthesis of ATP 39
2.3.1 Chloroplasts and Mitochondria Exhibit Specific Compartments 39
2.3.2 Chloroplasts and Mitochondria Synthesize ATP by Chemiosmosis 41
Summary 43
Chapter Review 43
Further Reading 43
Chapter 3 · The Dual Role of Sunlight:Energy and Information 45
3.1 The Physical Nature of Light 45
3.1.1 Light Is Electromagnetic Energy Which Exists in Two Forms 45
3.1.2 Light Can Be Characterized as a Wave Phenomenon 46
3.1.3 Light Can Be Characterized as a Stream of Discrete Particles 46
3.1.4 Light Energy Can Interact with Matter 47
3.1.5 How Does One Illustrate the Efficiency of Light Absorption and Its Physiological Effects 49
3.1.6 Accurate Measurement of Light Is Important in Photobiology 50
3.2 The Natural Radiation Environment 51
3.3 Photoreceptors Absorb Light for Use in a Physiological Process 52
3.1.1 Chlorophylls Are Primarily Responsible for Harvesting Light Energy for Photosynthesis 52
3.3.2 Phycobilins Serve as Accessory Light-Harvesting Pigments in the Red Algae and Cyanobacteria or as a Critical Regulatory System in Green Plants 54
3.3.3 Carotenoids Account for the Autumn Colors 55
3.3.4 Cryptochrome Is a Photoreceptor Sensitive to Blue and UV-A Light 57
3.3.5 UV-B Radiation May Act as a Developmental Signal 57
3.3.6 Flavonoids Provide the Myriad of Flower Colors and Act as a Natural Sunscreen 58
3.3.7 Betacyanins and Beets 60
Summary 60
Chapter Review 61
Further Reading 61
Chapter 4 · Energy Conservation in Photosynthesis: Harvesting Sunlight 63
4.1 Leaves Are Photosynthetic Machines Which Maximize the Absorption of Light 64
4.2 Photosynthesis Is an Oxidation-Reduction Process 66
4.3 Photosynthetic Electron Transport 68
4.3.1 Photosystems Are Major Components of the Photosynthetic Electron Transport Chain 68
4.3.2 Photosystem Ⅱ Oxidizes Water to Produce Oxygen 71
4.3.3 The Cytochrome Complex and Photosystem ⅠOxidize Plastoquinol 72
4.4 Photophosphorylation Is the Light-Dependent Synthesis of ATP 73
4.5 Lateral Heterogeneity Is the Unequal Distribution of Thylakoid Complexes 75
4.6 Light-Harvesting Complexes Are Superantenna Complexes that Regulate Energy Distribution 76
4.7 Photoinhibition of Photosynthesis: Photoprotection versus Photodamage 78
4.7.1 Carotenoids Serve a Dual Function: Light Harvesting and Photoprotection 78
4.7.2 Oxygen May Protect against Photoinhibition by Acting as an Alternative Electron Acceptor 80
4.7.3 The D1 Repair Cycle Overcomes Photodamage to PSII 82
4.8 Inhibitors of Photosynthetic Electron Transport Are Effective Herbicides 83
Summary 86
Chapter Review 86
Fur ther Reading 87
Box 4.1 · Historical Perspective—The Discovery of Photosynthesis 66
Box 4.2 · The Case for Two Photosystems 84
Chapter 5 · Energy Conservation in Photosynthesis: CO2 Assimilation 89
5.1 Stomatal Complex Controls Leaf Gas Exchange and Water Loss 90
5.2 CO2 Enters the Leaf by Diffusion 92
5.3 How Do Stomata Open and Close? 94
5.4 Stomatal Movements Are Also Controlled By External Environmental Factors 96
5.4.1 Light and Carbon Dioxide Regulate Stomatal Opening 96
5.4.2 Water Status and Temperature Influence Stomatal Opening 98
5.4.3 Stomatal Movements Follow Endogenous Rhythms 98
5.5 The Photosynthetic Carbon Reduction (PCR) Cycle 98
5.5.1 The PCR Cycle Reduces CO2 to Produce a 3-Carbon Sugar 98
5.5.2 The Carboxylation Reaction Fixes the CO2 99
5.5.3 ATP and NADPH Are Consumed in the PCR Cycle 102
5.5.4 What Are the Energetics of the PCR Cycle? 102
5.6 The PCR Cycle Is Highly Regulated 102
5.6.1 The Regeneration of RuBP Is Autocatalytic 102
5.6.2 Rubisco Activity Is Regulated Indirectly by Light 103
5.6.3 Other PCR Enzymes Are also Regulated by Light 104
5.7 Chloroplasts of C3 Plants also Exhibit Competing Carbon Oxidation Processes 104
5.7.1 Rubisco Catalyzes the Fixation of Both CO2 and O2 105
5.7.2 Why Photorespiration? 106
5.7.3 In Addition to PCR, Chloroplasts Exhibit an Oxidative Pentose Phosphate Cycle (OPPC) 108
5.8 The C4 Syndrome: Another Biochemical Mechanism to Assimilate CO2 108
5.9 The C4 Syndrome Is Usually Associated with Kranz Leaf Anatomy 112
5.10 The C4 Syndrome Has Ecological Significance 112
5.10 .1 The C4 Syndrome Is Differentially Sensitive to Temperature 113
5.10 .2 The C4 Syndrome Is Associated with Water Stress 113
5.11 Crassulacean Acid Metabolism (CAM): An Adaptation to Life in the Desert 115
5.11 .1 Is CAM a Variation of the C4 Syndrome? 115
5.11 .2 CAM Plants Are Particularly Suited to Dry Habitats 115
5.12 C4 and CAM Photosynthesis Require Precise Regulation and Temporal Integration 117
Summary 121
Chapter Review 122
Further Reading 122
Box 5.1 · Enzymes 118
Chapter 6 · Allocation, Translocation, and Partitioning of Photoassimilates 123
6.1 Starch and Sucrose Are Biosynthesized in Two Different Compartments 124
6.1.1 Starch Is Biosynthesized in the Stroma 124
6.1.2 Sucrose Is Biosynthesized in the Cytosol 125
6.2 Starch and Sucrose Biosynthesis Are Competitive Processes 126
6.3 Photoassimilates Are Translocated over Long Distances 128
6.3.1 What Is the Composition of the Photoassimilate Translocated by the Phloem? 129
6.4 Sieve Elements Are the Principal Cellular Constituents of the Phloem 131
6.4.1 Phloem Exudate Contains a Significant Amount of Protein 132
6.5 Direction of Translocation Is Determined by Source-Sink Relationships 133
6.6 Phloem Translocation Occurs by Mass Transfer 133
6.7 Phloem Loading and Unloading Regulate Translocation and Partitioning 136
6.7.1 Phloem Loading Can Occur Symplastically or Apoplastically 136
6.7.2 Phloem Unloading May Occur Symplastically or Apoplastically 138
6.8 Photoassimilate Is Distributed Between Different Metabolic Pathways and Plant Organs 139
6.8.1 Photoassimilates May Be Allocated to a Variety of Metabolic Functions in the Source or the Sink 140
6.8.2 Distribution of Photoassimilates Between Competing Sinks Is Determined By Sink Strength 141
6.9 Xenobiotic Agrochemicals Are Translocated in the Phloem 143
Summary 144
Chapter Review 144
Further Reading 144
Chapter 7 · Cellular Respiration: Unlocking the Energy Stored in Photoassimilates 145
7.1 Cellular Respiration Consists of a Series of Pathways by Which Photoassimilates Are Oxidized 146
7.2 Sucrose and Starch Are Broken Down into Glucose 147
7.2.1 α-Amylase Produces Maltose and Limit Dextrins 148
7.2.2 β-Amylase Produces Maltose 148
7.2.3 Limit Dextrinase Is a Debranching Enzyme 148
7.2.4 α-Glucosidase Hydrolyzes Maltose 148
7.2.5 Starch Phosphorylase Catalyzes the Phosphorolytic Degradation of Starch 149
7.3 Glycolysis Converts Sugars to Pyruvic Acid 150
7.3.1 Hexoses Must Be Phosphorylated to Enter Glycolysis 150
7.3.2 Triose Phosphates Are Oxidized to Pyruvate 151
7.4 The Oxidative Pentose Phosphate Pathway Is an Alternative Route for Glucose Metabolism 151
7.5 The Fate of Pyruvate Depends on the Availability of Molecular Oxygen 152
7.6 Oxidative Respiration Is Carried Out by the Mitochondrion 153
7.6.1 In the Presence of Molecular Oxygen, Pyruvate Is Completely Oxidized to CO2 and Water by the Citric Acid Cycle 153
7.6.2 Electrons Removed from Substrate in the Citric Acid Cycle Are Passed to Molecular Oxygen Through the Mitochondrial Electron Transport Chain 154
7.7 Energy Is Conserved in the Form of ATP in Accordance with Chemiosmosis 156
7.8 Plants Contain Several Alternative Electron Pathways 157
7.8.1 Plant Mitochrondria Contain External Dehydrogenases 157
7.8.2 Plants Have a Rotenone-Insensitive NADH Dehydrogenase 158
7.8.3 Plants Exhibit Cyanide-Resistant Respiration 158
7.9 Many Seeds Store Carbon as Oils Which Are Converted to Sugar 159
7.10 Respiration Provides Carbon Skeletons for Biosynthesis 161
7.11 Respiration Rate Varies with Development and Metabolic State 162
7.12 Respiration Rate Responds to Environmental Conditions 163
7.12 .1 Light 163
7.12 .2 Temperature 164
7.12 .3 Oxygen Availability 164
Summary 165
Chapter Review 165
Further Reading 165
Chapter 8 · Nitrogen Assimilation 167
8.1 The Nitrogen Cycle: A Complex Pattern of Exchange 167
8.1.1 Ammonification, Nitrification, and Denitrification Are Essential Processes in the Nitrogen Cycle 168
8.1.2 Nitrogen Fixation Reduces N2 to Ammonia 168
8.2 Biological Nitrogen Fixation Is Exclusively Prokaryotic 169
8.2.1 Some Nitrogen-Fixing Bacteria Are Free-Living Organisms 169
8.2.2 Symbiotic Nitrogen Fixation Involves Specific Associations Between Bacteria and Plants 169
8.3 Legumes Exhibit Symbiotic Nitrogen Fixation 170
8.3.1 Rhizobia Infect the Host Roots Which Induces Nodule Development 170
8.4 The Biochemistry of Nitrogen Fixation 174
8.4.1 Nitrogen Fixation Is Catalyzed by the Enzyme Dinitrogenase 174
8.4.2 Nitrogen Fixation Is Energetically Costly 175
8.4.3 Dinitrogenase Is Sensitive to Oxygen 175
8.4.4 Dinitrogenase Results in the Production of Hydrogen Gas 176
8.5 The Genetics of Nitrogen Fixation 177
8.5.1 nif Genes Code for Nitrogenase 177
8.5.2 nod Genes and nif Genes Regulate Nodulation 177
8.5.3 What Is the Source of Heme for Leghemoglobin? 177
8.6 NH3 Produced by Nitrogen Fixation is Converted to Organic Nitrogen 178
8.6.1 Ammonium Is Assimilated by GS/GOGAT 178
8.6.2 Fixed Nitrogen Is Exported As Asparagine and Ureides 179
8.7 Plants Generally Take up Nitrogen in the Form of Nitrate 181
8.8 Nitrogen Cycling: Simultaneous Importand Export 182
8.9 Agricultural and Ecosystem Productivity Is Dependent on Nitrogen Supply 183
Summary 184
Chapter Review 185
Further Reading 185
Box 8.1 · Lectins—Proteins with a Sweet Tooth 172
Chapter 9 · Carbon Assimilation and Productivity 187
9.1 Productivity Refers to an Increase in Biomass 187
9.2 Carbon Economy Is Dependent on the Balance Between Photosynthesis and Respiration 188
9.3 Productivity Is Influenced by a Variety of Genetic and Environmental Factors 189
9.3.1 Fluence Rate 189
9.3.2 Available CO2 190
9.3.3 Temperature 192
9.3.4 Soil Water Potential 193
9.3.5 Nutrient Supply, Pathology, and Pollutants 193
9.3.6 Leaf Factors 194
9.4 Primary Productivity on a Global Scale 196
Summary 197
Chapter Review 197
Further Reading 198
Part 2 · Plants, Water, and Minerals 200
Chapter 10 · Plant Cells and Water 201
10.1 Water Has Unique Physical and Chemical Properties 202
10.2 The Thermal Properties of Water Are Biologically Important 203
10.2.1 Water Exhibits a Unique Thermal Capacity 203
10.2.2 Water Exhibits a High Heat of Fusion and Heat of Vaporization 203
10.3 Water Is the Universal Solvent 204
10.4 Polarity of Water Molecules Results in Cohesion and Adhesion 205
10.5 Water Movement May Be Governed by Diffusion or by Bulk Flow 205
10.5.1 Bulk Flow Is Driven by Hydrostatic Pressure 205
10.5.2 Fick's First Law Describes the Process of Diffusion 206
10.6 Osmosis Is the Diffusion of Water Across a Selectively Permeable Membrane 207
10.6.1 Osmosis in Plant Cells Is Indirectly Energy Dependent 207
10.6.2 The Chemical Potential of Water Has an Osmotic as Well as Pressure Component 209
10.7 Hydrostatic Pressure and Osmotic Pressure Are Two Components of Water Potential 210
10.8 Water Potential Is the Sum of Its Component Potentials 211
10.9 Dynamic Flux of H2O Is Associated with Changes in Water Potential 212
10.10 How Elastic Are Cell Walls? 213
Summary 217
Chapter Review 217
Further Reading 217
Box 10.1 · Osmosensors 214
Chapter 11 · Whole Plant Water Relations 219
11.1 Transpiration Is Driven by Differences in Vapor Pressure 220
11.2 Transpiration Can Be Measured by Weight Loss and Gas Exchange 221
11.3 The Driving Force of Transpiration Is Differences in Vapor Pressure 221
11.4 The Rate of Transpiration Is Influenced by Environmental Factors 222
11.4.1 What Are the Effects of Humidity? 223
11.4.2 What Is the Effect of Temperature? 224
11.4.3 What Is the Effect of Wind? 224
11.5 Water Conduction Occurs via Tracheary Elements 225
11.6 The Ascent of Xylem Sap Is Explained by Combining Transpiration with Cohesive Forces of Water 228
11.6.1 Root Pressure Is Related to Root Structure 229
11.6.2 Water Rise by Capillarity Is Due to Adhesion and Surface Tension 231
11.6.3 The Cohesion Theory Best Explains the Ascent of Xylem Sap 231
11.7 Water Loss Due to Transpiration Must Be Replenished 235
11.7.1 Soil Is a Complex Medium 235
11.8 Roots Absorb and Transport Water 237
11.9 The Permeability of Roots to Water Varies 237
11.10 Radial Movement of Water Through the Root Involves Two Possible Pathways 238
Summary 240
Chapter Review 240
Further Reading 240
Box 11.1 · Why Transpiration? 226
Box 11.2 · Forces Involved in Capillary Rise 232
Chapter 12 · Plants and Inorganic Nutrients 241
12.1 Methods and Nutrient Solutions 242
12.1.1 Interest in Plant Nutrition Is Rooted in the Study of Agriculture and Crop Productivity 242
12.1.2 The Use of Hydroponic Culture Helped to Define the Mineral Requirements of Plants 242
12.1.3 Modern Techniques Overcome Inherent Disadvantages of Simple Solution Culture 243
12.2 The Essential Nutrient Elements 245
12.2.1 Seventeen Elements Are Deemed to Be Essential for Plant Growth and Development 245
12.2.2 The Essential Nutrients Are Generally Classed as either Macronutrients or Micron Nutrients 245
12.2.3 Determining Essentiality of Micronutrients Presents Special Problems 245
12.3 Beneficial Elements 246
12.3.1 Sodium Is an Essential Micronutrient for C4 Plants 247
12.3.2 Silicon May Be Beneficial for a Variety of Species 247
12.3.3 Cobalt Is Required by Nitrogen-Fixing Bacteria 247
12.3.4 Some Plants Tolerate High Concentrations of Selenium 247
12.4 Nutrient Functions and Deficiency Symptoms 247
12.4.1 A Plant's Requirement for a Particular Element Is Defined in Terms of Critical Concentration 248
12.4.2 Nitrogen Is a Constituent of Many Critical Macromolecules 249
12.4.3 Phosphorous Is Part of the Nucleic Acid Backbone and Has a Central Function in Intermediary Metabolism 249
12.4.4 Potassium Activates Enzymes and Functions in Osmoregulation 250
12.4.5 Sulfur Is an Important Constituent of Proteins,Coenzymes, and Vitamins 250
12.4.6 Calcium Is Important in Cell Division, Cell Adhesion, and as a Secondary Messenger 251
12.4.7 Magnesium Is a Constituent of the Chlorophyll Molecule and Is an Important Regulator of Enzyme Reaction 251
12.4.8 Iron Is Required for Chlorophyll Synthesis and Electron Transfer Reactions 251
12.4.9 Boron Appears to Have a Role in Cell Division and Elongation and Contributes to the Structural Integrity of the Cell Wall 253
12.4.10 Copper Is a Necessary Cofactor for Oxidative Enzymes 254
12.4.11 Zinc Is an Activator of Numerous Enzymes 254
12.4.12 Manganese Is an Enzyme Cofactor as Well as Part of the Oxygen-Evolving Complex in the Chloroplast 254
12.4.13 Molybdenum Is a Key Component of Nitrogen Metabolism 254
12.4.14 Chlorine Has a Role in Photosynthetic Oxygen Evolution and Balances Charge Across Cellular Membranes 255
12.4.15 The Role of Nickel Is Not Clear 255
12.5 Toxicity of Micronutrients 256
Summary 256
Chapter Review 256
Further Reading 257
Chapter 13 · Roots, Soils, and Nutrient Uptake 259
13.1 The Soil as a Nutrient Reservoir 260
13.1.1 Colloids Are a Significant Component of Most Soils 260
13.1.2 Colloids Present a Large, Negatively Charged Surface Area 260
13.1.3 Soil Colloids Reversibly Adsorb Cations from the Soil Solution 261
13.1.4 The Anion Exchange Capacity of Soil Colloids Is Relatively Low 26113.2 Nutrient Uptake 262
13.2.1 Nutrient Uptake by Plants Requires Transport of the Nutrient across Root Cell Membranes 262
13.2.2 Simple Diffusion Is a Purely Physical Process 262
13.2.3 The Movement of Most Solutes across Membranes Requires the Participation of Specific Transport Proteins 263
13.2.4 Active Transport Requires the Expenditure of Metabolic Energy 263
13.3 Selective Accumulation of Ions by Roots 266
13.4 Electrochemical Gradients and Ion Movement 266
13.4.1 Ions Move in Response to Electrochemical Gradients 266
13.4.2 The Nernst Equation Helps to Predict Whether an Ion Is Exchanged Actively or Passively 267
13.5 Active Transport and Electrogenic Pumps 269
13.5.1 Active Transport Is Driven by ATPase-Proton Pumps 269
13.5.2 The ATPase-Proton Pumps of Plasma Membranes and Vacuolar Membranes Are Different 270
13.5.3 K+ Exchange Is Mediated by Two Classes of Transport Proteins 271
13.6 Ion Uptake by Roots 272
13.6.1 A First Step in Mineral Uptake by Roots Is Diffusion into the Apparent Free Space 272
13.6.2 Apparent Free Space Is Equivalent to the Apoplast of the Root Epidermal and Cortical Cells 273
13.7 The Radial Path of Ion Movement Through Roots 274
13.7.1 Ions Entering the Stele Must First Be Transported from the Apparent Free Space into the Symplast 274
13.7.2 Ions Are Actively Secreted into the Xylem Apoplast 274
13.7.3 Emerging Secondary Roots May Contribute to the Uptake of Some Solutes 275
13.8 Root-Microbe Interactions 276
13.8.1 Bacteria Other Than Nitrogen Fixers Contribute to Nutrient Uptake by Roots 276
13.8.2 Mycorrhizae Are Fungi that Increase the Volume of the Nutrient Depletion Zone Around Roots 276
Summary 279
Chapter Review 279
Further Reading 280
Box 13.1 · Electrophysiology—Exploring Ion Channels 264
Part 3 · Plant Development 281
Chapter 14 · Patterns in Plant Development 283
14.1 Growth, Differentiation, and Development 283
14.1.1 Development Is the Sum of Growth and Differentiation 283
14.1.2 Growth Is an Irreversible Increase in Size 284
14.1.3 Differentiation Refers to Qualitative Changes That Normally Accompany Growth 284
14.2 Control of Development 285
14.2.1 The Orderly Development of a Plant Requires a Programmed Sequence of Gene Expression 285
14.2.2 Hormones Coordinate Cell-Cell Interactions 287
14.2.3 A Continuous Stream of Environmental Signals Provide Information That Plants Use to Modify Their Development 287
14.3 Signal Perception and Transduction 287
14.3.1 Signals Are Perceived by Protein Receptors 288
14.3.2 Signal Transduction Includes a Diverse Array of Second Messengers and Biochemical Mechanisms 288
14.3.3 There Is Extensive Cross-Talk among Signal Pathways 291
14.4 Cell Walls and Cell Growth 291
14.4.1 Cell Growth Is Driven by Water Uptake and Limited by the Strength and Rigidity of the Cell Wall 291
14.4.2 Extension of the Cell Wall Requires Wall-Loosening Events That Enable Load-Bearing Elements in the Wall to Yield to Turgor Pressure 292
14.4.3 Wall Loosening and Cell Expansion Are Stimulated by Low pH and Expansins 293
14.5 A Survey of Plant Development 294
14.5.1 Seed Structure and Development 294
14.5.2 Seed Germination 295
14.5.3 Shoot Development 295
14.5.4 Root Development 299
14.5.5 Flower Evocation and Development 301
14.5.6 Flower and Fruit Development 301
14.5.7 Senescence and Programmed Cell Death Are the Final Stages of Development 302
14.6 Kinetic Analysis of Growth 303
14.6.1 Growth of Microorganisms in Culture Exhibit Exponential Growth 303
14.6.2 Growth of Multicellular Organisms Is Determined by the Activity of the Meristem 306
Summary 306
Chapter Review 306
Further Reading 306
Box 14.1 · Development in a Mutant Weed 286
Box 14.2 · Ubiquitin and Proteosomes—Cleaning Up Unwanted Proteins 304
Chapter 15 · The Plant Hormones:Biochemistry and Metabolism 309
15.1 Do Plants Have Hormones? 309
15.1.1 There Are Subtle Differences Between Animal and Plant Hormones 310
15.1.2 The List of Plant Hormones Is Growing 312
15.1.3 The Amount of Hormone in a Tissue Is Governed by Several Factors 312
15.2 Auxin 313
15.2.1 The Principal Auxin in Plants Is Indole-3-Acetic Acid (IAA) 314
15.2.2 IAA Is Synthesized from the Amino Acid L-Tryptophan 316
15.2.3 Some Plants Do Not Require Tryptophan for IAA Biosynthesis 316
15.2.4 IAA May Be Stored As Inactive Conjugates 318
15.2.5 There Are Two Principal Mechanisms for Deactivation of IAA 318
15.3 Gibberellins 320
15.3.1 There Are Three Principal Sites for Gibberellin Biosynthesis 320
15.3.2 Gibberellins Are Terpenes, Sharing a Core Pathway with Several Other Hormones and a Wide Range of Secondary Products 322
15.3.3 Gibberellins Are Synthesized from Geranylgeranyl Pyrophosphate (GGPP) 323
15.3.4 Growth Retardants Block the Synthesis of Gibberellins 324
15.3.5 Gibberellins Are Deactivated by 2β-Hydroxylation 324
15.3.6 Gibberellin Transport Is Poorly Understood 325
15.4 Cytokinins 325
15.4.1 Cytokinins Are Synthesized Primarily in the Root 325
15.4.2 Cytokinin Biosynthesis Begins with the Condensation of an Isopentenyl Group with the Amino Group of Adenosine Monophosphate 326
15.4.3 Cytokinins May Be Reversibly or Irreversibly Deactivated by Conjugation and Irreversibly Deactivated by Oxidation 329
15.5. Abscisic Acid 329
15.5.1 Abscisic Acid Is Synthesized Primarily in Mature Leaves 330
15.5.2 Abscisic Acid Is Synthesized from the Cleavage Product of a 40-Carbon Carotenoid Precursor 330
15.5.3 Abscisic Acid Is Degraded by Oxidation to PhaseicAcid 332
15.6 Ethylene 332
15.6.1 Ethylene Is Synthesized from the Amino Acid Methionine 332
15.6.2 Ethylene and Polyamine Biosynthesis Share a Common Precursor 334
15.6.3 Excess Ethylene Is Subject to Oxidation 334
15.7 Brassinosteroids 334
15.7.1 Brassinosteroids Are Polyhydroxylated Sterols Derived from the Triterpene Squalene 335
15.7.2 Several Routes for Deactivation of Brassinosteroids Have Been Identified 335
15.8 Polyamines 335
15.8.1 The Pathway for Polyamine Biosynthesis Is the Same in Plants, Microorganisms, and Mammals 337
Summm y 338
Chapter Review 338
FurtherReading 339
Box 15.1 · Historical Perspectives—Discovering Plant Hormones 311
Chapter 16 · The Plant Hormones: Control of Development 341
16.1 Cell Division, Enlargement, and Differentiation 341
16.1.1 Cytokinins Are a Significant Factor in Regulating Cell Division 341
16.1.2 Cytokinins Regulate Progression Through the Cell Cycle 342
16.1.3 Auxins Stimulate Cell Enlargement in Excised Tissues 344
16.1.4 The Acid-Growth Hypothesis Explains Auxin Control of Cell Enlargement 344
16.1.5 Maintenance of Auxin-Induced Growth Requires Gene Activation 347
16.1.6 Many Aspects of Plant Development Are Linked to the Polar Transport of Auxin 349
16.1.7 Auxins and Cytokinins Regulate Vascular Differentiation 351
16.2 Seed Development and Germination 352
16.2.1 The Level and Activities of Various Hormones Change Dramatically During Seed Development 352
16.2.2 Gibberellins Stimulate Mobilization of Nutrient Reserves During Germination of Cereal Grains 353
16.3 Shoot and Root Development 356
16.3.1 Gibberellins Stimulate Hyperelongation of Intact Stems, Especially in Dwarf and Rosette Plants 356
16.3.2 Inhibition of Gibberellin Biosynthesis Has Commercial Applications 357
16.3.3 Hormone Mutants Indicate a Role for Brassinosteroids and Ethylene in Stem Growth 358
16.3.4 The Ratio of Auxin to Cytokinin Controls the Growth of Axillary Buds 358
16.3.5 Root Elongation and Development Is Particularly Sensitive to Auxin and Ethylene 359
16.4 Senescence and Abscission 360
16.4.1 Cytokinins and Ethylene Are Antagonistic in the Regulation of Nutrient Mobilization and Senescence 360
16.4.2 Auxin Regulates Leaf Abscission 362
16.5 Flower and Fruit Development 362
16.5.1 Gibberellins Promote Precocious Flowering in Some Species 363
16.5.2 Auxin and Gibberellin Influence the Sex of Flowers 363
16.5.3 Hormones Influence Fruit Set and Development 364
16.6 Ethylene 364
16.6.1 The Study of Ethylene Presents a Unique Set of Problems 364
16.6.2 Ethylene Affects Many Aspects of Vegetative Development 364
Summary 365
Chapter Review 366
Further Reading 366
Box 16.1 · The Cell Cycle and Control of Cell Division 343
Box 16.2 · Commercial Applications of Hormones 348
Chapter 17 · Photomorphogenesis:Responding to Light 367
17.1 Photomorphogenesis 368
17.2 Phytochrome: Responses to Red and Far-Red Light 368
17.2.1 Photoreversibility Is the Hallmark of Phytochrome Action 370
17.2.2 Phytochromes Are Phycobilin Pigments 371
17.2.3 Conversion of Pr to Pfr in Etiolated Seedlings Leads to a Loss of Both Pfr and Total Phytochrome 372
17.3 Phytochrome Responses Can Be Grouped According to Their Fluence Requirements 374
17.3.1 The Most Studied Low Fluence Responses (LFRs) Are De-etiolation and Seed Germination 374
17.3.2 Very Low Fluence Responses Are Not Photoreversible 376
17.3.3 High Irradiance Reactions Require Prolonged Exposure to Relatively High Fluence Rates 377
17.4 Phytochrome under Natural Conditions 377
17.4.1 PhA May Function to Detect the Presence of Light 378
17.4.2 Phytochrome Detects Canopy Shading and End-of-Day Signals 378
17.5 Responses to Blue and UV-A Light 381
17.5.1 Cryptochrome Is a Flavoprotein 381
17.5.2 Phototropin Is a Blue Light-Dependent Kinase 382
17.5.3 A Hybrid Blue-Light Photoreceptor Has Been Isolated from a Fern 382
17.6 De-etiolation in Arabidopsis. A Case Study in Photoreceptor Interactions 383
17.7 Photoreceptor Signal Transduction 384
17.7.1 Phytochromes Have Kinase Activity 384
17.7.2 Pfr Regulates Gene Expression 384
17.7.3 Phytochrome May Migrate from the Cytoplasm to the Nucleus 386
17.8 Some Plant Responses Are Regulated by UV-B Light 387
Summary 387
Chapter Review 388
Further Reading 388
Box 17.1 · Historical Perspectives: The Discovery of Phytochrome 369
Chapter 18 · Plant Movements—Orientation in Space 391
18.1 Phototropism 392
18.1.1 Phototropism Is a Response to a Light Gradient across an Organ 392
18.1.2 Phototropism in Coleoptiles Is Mediated by a Flavoprotein 393
18.1.3 Fluence Response Curves Illustrate the Complexity of Phototropic Responses 394
18.1.4 The Phototropic Response Is Attributed to a Lateral Redistribution of Diffusible Auxin 395
18.2 Gravitropism 398
18.2.1 Gravitropism Is More Than Simply Up and Down 398
18.2.2 The Gravitational Stimulus Is the Product of Intensity and Time 399
18.2.3 In Roots, Gravity Is Perceived in the Root Cap 401
18.2.4 The Sedimentation of Starch-Filled Amyloplasts Is an Initial Gravity-Sensing Event 402
18.2.5 Gravitropism, Like Phototropism, Is an Auxin-Dependent Differential Growth Response 403
18.2.6 The Gravitropic Signal Transduction Chain May Involve Stretch-Activated Ion Channels, pH Changes in the Root Cap, and Redistribution of Calcium Ions 405
18.2.7 Gravitropism in Grass Stems Occurs in the False Pulvinus 407
18.2.8 Plants Follow Different Rules in the Microgravity Environment of Space 408
18.3 Nastic Movements 408
18.3.1 Nyctinastic Movements Are Rhythmic Movements Involving Reversible Turgor Changes 408
18.3.2 Nvctinastic Movements Are Due to Ion Fluxes and Resulting Osmotic Responses in Specialized Motor Cells 409
18.3.3 Seismonasty Is a Response to Mechanical Stimulation 412
Summary 413
Chapter Review 414
Further Reading 414
Box 18.1 · Methods in the Study of Gravitropism 400
Chapter 19 · Measuring Time: The Control of Development by Photoperiod and Endogenous Clocks 415
19.1 Photoperiodism 416
19.1.1 Photoperiodic Responses May Be Characterized By a Variety of Response Types 416
19.1.2 Critical Daylength Defines Short-Day and Long-Day Responses 417
19.1.3 Plants Actually Measure the Length of the Dark Period 419
19.1.4 The Photoperiodic Signal Is Perceived by the Leaves 420
19.1.5 Phytochrome Is the Principal Photoreceptor for Photoperiodism 421
19.1.6 Photoperiodism Normally Requires a Period of High Fluence Light Before or After the Dark Period 422
19.1.7 Three Different Hypotheses Have Been Proposed to Account for the Floral Stimulus 422
19.1.8 Photoperiodic Behavior Is Often Modified by Temperature 423
19.2 The Biological Clock 426
19.2.1 Clock-Driven Rhythms Persist under Constant Conditions 426
19.2.2 The Circadian Clock Is Temperature-Compensated 428
19.2.3 Light Resets the Biological Clock on a Daily Basis 428
19.2.4 The Circadian Clock Is a Significant Component in Photoperiodic Time Measurement 429
19.2.5 Several Clock-Associated Genes Have Been Identified 431
19.2.6 The Circadian Clock in Insects, Animals, and Cyanobacteria Is a Negative Feedback Loop 432
19.3 Floral Induction 433
19.3.1 Flower Initiation and Development Involves the Sequential Action of Three Sets of Genes 433
19.3.2 Flowering Time Genes Influence the Duration of Vegetative Growth 434
19.3.3 Floral Identity Genes and Organ Identity Genes Overlap in Time and Function 435
19.4 Photoperiodism in Nature 436
Summary 438
Chapter Review 438
Further Reading 439
Box 19.1 · Historical Perspectives: The Discovery of Photoperiodism 416
Box 19.2 · Historical Perspectives: The Biological Clock 424
Chapter 20 · Temperature: Plant Development and Distribution 441
20.1 Temperature in the Plant Environment 441
20.2 Temperature and Flowering Response 443
20.2.1 Vernalization Occurs Most Commonly in Winter Annuals and Biennials 443
20.2.2 The Effective Temperature for Vernalization Is Variable 444
20.2.3 The Vernalization Treatment Is Perceived by the Shoot Apex 445
20.2.4 The Vernalized State Is Transmissible 445
20.2.5 Gibberellin and Vernalization Operate Through Independent Genetic Pathways 446
20.3 Bud Dormancy 446
20.3.1 Bud Dormancy Is Induced Primarily by Photoperiod 447
20.3.2 Temperature Is a Significant Factor in Breaking Bud Dormancy 447
20.4 Seed Dormancy and Germination 448
20.4.1 Numerous Factors Influence Seed Dormancy and Germination 448
20.4.2 Temperature Has a Significant Impact on Seed Dormancy 449
20.5 Responses to Change in Temperature 450
20.6 Influence of Temperature on Growth and Plant Distribution 451
20.6.1 Coasts and Deserts: A Case Study 452
20.6.2 Temperature Influences the Distribution of C3 and C4 Grasses on Mountain Slopes 454
Summary 454
Chapter Review 455
Further Reading 455
Part 4 · Stress and Secondary Metabolism 457
Chapter 21 · Plant Environmental Stress Physiology 459
21.1 What Is Plant Stress? 459
21.2 Plants Respond to Stress in Several DifferentWays 460
21.3 Abiotic Stress 461
21.3.1 Water Stress Is a Persistent Threat to Plant Survival 461
21.3.2 Water Stress Leads to Membrane Damage 462
21.3.3 Photosynthesis Is Particularly Sensitive to Water Stress 462
21.3.4 Stomata Respond to Water Deficit 463
21.3.5 Osmotic Adjustment Is a Response to Water Stress 465
21.3.6 Water Deficit Affects Shoot and Root Growth 466
21.3.7 Water Stress May Induce a Decrease in Leaf Area 467
21.4 Temperature Stress 467
21.4.1 Many Plants Are Chilling Sensitive 468
21.4.2 North Temperate Overwintering Plants Survive Freezing Stress 468
21.4.3 Cold Acclimation Increases Resistance to Freezing Stress 470
21.4.4 Cold Acclimation and Freezing Tolerance in Herbaceous Species Is a Complex Interaction Between Light and Low Temperature 471
21.4.5 High Temperature Stress Is a Major Factor in Plant Productivity 472
21.5 Salt Stress, Water Deficits and Ion Toxicity 474
21.6 Pollution Represents a Relatively New Abiotic Stress 476
21.6.1 Heavy Metals 476
21.6.2 Air Pollution 477
21.7 Insects and Disease Represent Potential Biotic Stresses 479
21.7.1 Hypersensitive Reaction Is a Sensing/Signalling Mechanism Initiated by a Biotic Stress 479
21.7.2 How Do Plants Recognize Potential Pathogens and Initiate Defense Responses? 479
21.7.3 Systemic Acquired Resistance Represents a Plant Immune Response 480
21.7.4 Jasmonates Mediate Insect and Disease Resistance 481
Summary 490
Chapter Review 491
Further Reading 491
Box 21.1 · Monitoring Plant Stress by Chlorophyll Fluorescence 482
Box 21.2 · Ecophysiology, Plant Biomes, and Weather 484
Chapter 22 · Secondary Plant Metabolites 493
22.1 Primary and Secondary Metabolites 493
22.2 Terpenoids 494
22.2.1 The Terpenoids Are a Chemically and Functionally Diverse Group of Molecules That Share a Common Biosynthetic Pathway 494
22.2.2 Many Terpenoids Are Active against Insect Herbivory 495
22.2.3 Steroids and Sterols Are Tetracyclic Triterpenoids 497
22.2.4 Polyterpenes Include the Carotenoid Pigments and Natural Rubber 497
22.3 Glycosides 499
22.3.1 Saponins Are Terpene Glycosides with Detergent Properties 499
22.3.2 Cardian Glycosides Are Highly Toxic, Modified Steroid Glycosides 500
22.3.3 Cyanogenic Glycosides Are a Natural Source of Hydrogen Cyanide 501
22.3.4 Glucosinolates Are Sulfur-Containing Precursors to Mustard Oils 502
22.4 Phenylpropenoids 503
22.4.1 Shikimic Acid Is a Key Intermediate in the Synthesis of Aromatic Amino Acids and Phenylpropenoids 503
22.4.2 The Simplest Phenolic Molecules Are Essentially Deaminated Versions of the Corresponding Amino Acids 503
22.4.3 Coumarins and Coumarin Derivatives Function as Anticoagulants 506
22.4.4 Lignin Is a Major Structural Component of Secondary Cell Walls 507
22.4.5 Flavonoids and Stilbenes Have Parallel Biosynthetic Pathways 508
22.4.6 Tannins Denature Proteins and Provide an Astringent Taste to Foods 508
22.5 Alkaloids 510
22.5.1 Alkaloids Are a Large Family of Chemically Unrelated Molecules 510
22.5.2 Alkaloids Are Noted Primarily for Their Pharmacological Properties and Medical Applications 510
22.5.3 Like Many Other Secondary Metabolites,Alkaloids Serve as Preformed Chemical Defense Molecules 512
Summary 513
Chapter Review 513
Further Reading 513
Part 5 · Biotechnology 515
Chapter 23 · Biotechnology: Engineering Plants for the Future 517
23.1 Modern Biotechnology Is Synonymous with Recombinant DNA Technology 518
23.1.1 DNA Recombination Allows the Movement of Selected Genes Between Organisms 518
23.1.2 The Most Widely Used Vector for Introducing Foreign Genes into Plants is the Ti Plasmid of the Crown-Gall Bacterium Agrobacterium Tumefaciens 519
23.1.3 Electroporation and Biolistics Are Methods for Direct Delivery of DNA into Plant Cells 522
23.1.4 Genetic Engineering Is a New Chapter in the Long Histoy of Plant Breeding 522
23.2 Tissue and Cell Culture and Protoplast Fusion 523
23.2.1 The Culture of Plant Cells and Tissues Has Been Exploited Since the 1930s 523
23.2.2 Protoplasts Are Naked Plant Cells That Can Be Fused to Make Somatic Hybrids 523
23.2.3 Tissue Culture Has Made Possible Large-Scale Cloning of Plants 524
23.3 Plant Protection 524
23.3.1 Herbicide-Resistant Crops Encourage More Efficient Use of Herbicides 525
23.3.2 Herbicide Resistance Can Be Achieved by Overexpression of Tolerant Enzymes 525
23.3.3 Herbicide Resistance in Weeds Is a Potentially Undesirable Side-Effect of Herbicide Use 526
23.3.4 Several Strategies Are Available for Protection against Insects and Disease 527
23.4 Metabolic Engineering: Improving Yield and Nutrition 528
23.4.1 One Target of Biotechnology Is Improved Carbon Gain and Nitrogen Metabolism 528
23.4.2 Boosting Vitamin Content Is One Way to Improve the Nutritional Quality of Foods 529
23.4.3 Oilseed Crops May Be Engineered to Produce Healthier Edible Oils 529
23.5 Molecular Farming Uses Plants as Living Factories 530
23.5.1 Transgenic Plants May Provide a Low-Cost Delivery System for Vaccines 531
23.5.2 Plants Can Be Engineered to Produce Biodegradable Plastics 531
23.6 Plants Have Potential as an Alternative Source of Renewable Fuels 532
23.7 Plants Remain Useful as a Source of Secondary Products 533
Summary 533
Chapter Review 534
Further Reading 534
Box 23.1 · Engineering Plants with Their Own Genes 519
Appendix Ⅰ · Standard Amino Acids and Their Structures 535
Appendix Ⅱ · Measuring Water Potential and Its Components 539
Index 545