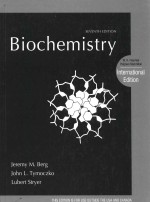
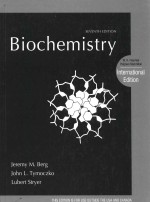
BIOCHEMISTRY INTERNATIONAL SEVENTH EDITIONPDF电子书下载
- 电子书积分:27 积分如何计算积分?
- 作 者:JEREMY M.BERG
- 出 版 社:PALGRAVE MACMILLAN
- 出版年份:2012
- ISBN:1429276355
- 页数:1098 页
Part Ⅰ THE MOLECULAR DESIGN OF LIFE 1
Chapter 1 Biochemistry:An Evolving Science 1
1.1 Biochemical Unity Underlies Biological Diversity 1
1.2 DNA Illustrates the Interplay Between Form and Function 4
DNA is constructed from four building blocks 4
Two single strands of DNA combine to form a double helix 5
DNA structure explains heredity and the storage of information 5
1.3 Concepts from Chemistry Explain the Properties of Biological Molecules 6
The double helix can form from its component strands 6
Covalent and noncovalent bonds are important for the structure and stability of biological molecules 7
The double helix is an expression of the rules of chemistry 10
The laws of thermodynamics govern the behavior of biochemical systems 11
Heat is released in the formation of the double helix 12
Acid base reactions are central in many biochemical processes 13
Acid-base reactions can disrupt the double helix 14
Buffers regulate pH in organisms and in the laboratory 15
1.4 The Genomic Revolution Is Transforming Biochemistry and Medicine 17
The sequencing of the human genome is a landmark in human history 17
Genome sequences encode proteins and patterns of expression 18
Individuality depends on the interplay between genes and environment 19
APPENDIX:Visualizing Molecular Structures Ⅰ:Small Molecules 21
Chapter 2 Protein Composition and Structure 25
2.1 Proteins Are Built from a Repertoire of 20 Amino Acids 27
2.2 Primary Structure:Amino Acids Are Linked by Peptide Bonds to Form Polypeptide Chains 33
Proteins have unique amino acid sequences specified by genes 35
Polypeptide chains are flexible yet conformationally restricted 36
2.3 Secondary Structure:Polypeptide Chains Can Fold into Regular Structures Such As the Alpha Helix,the Beta Sheet,and Turns and Loops 38
The alpha helix is a coiled structure stabilized by intrachain hydrogen bonds 38
Beta sheets are stabilized by hydrogen bonding between polypeptide strands 40
Polypeptide chains can change direction by making reverse turns and loops 42
Fibrous proteins provide structural support for cells and tissues 43
2.4 Tertiary Structure:Water-Soluble Proteins Fold into Compact Structures with Nonpolar Cores 45
2.5 Quaternary Structure:Polypeptide Chains Can Assemble into Multisubunit Structures 48
2.6 The Amino Acid Sequence of a Protein Determines Its Three-Dimensional Structure 49
Amino acids have different propensities for forming alpha helices,beta sheets,and beta turns 50
Protein folding is a highly cooperative process 52
Proteins fold by progressive stabilization of intermediates rather than by random search 52
Prediction of three-dimensional structure from sequence remains a great challenge 54
Some proteins are inherently unstructured and can exist in multiple conformations 54
Protein misfolding and aggregation are associated with some neurological diseases 55
Protein modification and cleavage confer new capabilities 57
APPENDIX:Visualizing Molecular Structures Ⅱ:Proteins 60
Chapter 3 Exploring Proteins and Proteomes 67
The proteome is the functional representation of the genome 68
3.1 The Purification of Proteins Is an Essential First Step in Understanding Their Function 68
The assay:How do we recognize the protein that we are looking for? 69
Proteins must be released from the cell to be purified 69
Proteins can be purified according to solubility,size,charge,and binding affinity 70
Proteins can be separated by gel electrophoresis and displayed 73
A protein purification scheme can be quantitatively evaluated 77
Ultracentrifugation is valuable for separating biomolecules and determining their masses 78
Protein purification can be made easier with the use of recombinant DNA technology 80
3.2 Amino Acid Sequences of Proteins Can Be Determined Experimentally 81
Peptide sequences can be determined by automated Edman degradation 82
Proteins can be specifically cleaved into small peptides to facilitate analysis 84
Genomic and proteomic methods are complementary 86
3.3 Immunology Provides Important Techniques with Which to investigate Proteins 86
Antibodies to specific proteins can be generated 86
Monoclonal antibodies with virtually any desired specificity can be readily prepared 88
Proteins can be detected and quantified by using an enzyme-linked immunosorbent assay 90
Western blotting permits the detection of proteins separated by gel electrophoresis 91
Fluorescent markers make the visualization of proteins in the cell possible 92
3.4 Mass Spectrometry Is a Powerful Technique for the Identification of Peptides and Proteins 93
The mass of a protein can be precisely determined by mass spectrometry 93
Peptides can be sequenced by mass spectrometry 95
Individual proteins can be identified by mass spectrometry 96
3.5 Peptides Can Be Synthesized by Automated Solid-Phase Methods 97
3.6 Three-Dimensional Protein Structure Can Be Determined by X-ray Crystallography and NMR Spectroscopy 100
X-ray crystallography reveals three-dimensional structure in atomic detail 100
Nuclear magnetic resonance spectroscopy can reveal the structures of proteins in solution 103
Chapter 4 DNA,RNA,and the Flow of Information 113
4.1 A Nucleic Acid Consists of Four Kinds of Bases Linked to a Sugar-Phosphate Backbone 114
RNA and DNA differ in the sugar component and one of the bases 114
Nucleotides are the monomeric units of nucleic acids 115
DNA molecules are very long 117
4.2 A Pair of Nucleic Acid Chains with Complementary Sequences Can Form a Double-Helical Structure 117
The double helix is stabilized by hydrogen bonds and van der Waals interactions 117
DNA can assume a variety of structural forms 119
Z-DNA is a left-handed double helix in which backbone phosphates zigzag 120
Some DNA molecules are circular and supercoiled 121
Single-stranded nucleic acids can adopt elaborate structures 121
4.3 The Double Helix Facilitates the Accurate Transmission of Hereditary Information 122
Differences in DNA density established the validity of the semiconservative-replication hypothesis 123
The double helix can be reversibly melted 124
4.4 DNA Is Replicated by Polymerases That Take Instructions from Templates 125
DNA polymerase catalyzes phosphodiester-bridge formation 125
The genes of some viruses are made of RNA 126
4.5 Gene Expression Is the Transformation of DNA Information into Functional Molecules 127
Several kinds of RNA play key roles in gene expression 127
All cellular RNA is synthesized by RNA polymerases 128
RNA polymerases take instructions from DNA templates 130
Transcription begins near promoter sites and ends at terminator sites 130
Transfer RNAs are the adaptor molecules in protein synthesis 131
4.6 Amino Acids Are Encoded by Groups of Three Bases Starting from a Fixed Point 132
Major features of the genetic code 133
Messenger RNA contains start and stop signals for protein synthesis 134
The genetic code is nearly universal 135
4.7 Most Eukaryotic Genes Are Mosaics of Introns and Exons 135
RNA processing generates mature RNA 136
Many exons encode protein domains 137
Chapter 5 Exploring Genes and Genomes 145
5.1 The Exploration of Genes Relies on Key Tools 146
Restriction enzymes split DNA into specific fragments 147
Restriction fragments can be separated by gel electrophoresis and visualized 147
DNA can be sequenced by controlled termination of replication 149
DNA probes and genes can be synthesized by automated solid-phase methods 150
Selected DNA sequences can be greatly amplified by the polymerase chain reaction 151
PCR is a powerful technique in medical diagnostics,forensics,and studies of molecular evolution 152
The tools for recombinant DNA technology have been used to identify disease-causing mutations 153
5.2 Recombinant DNA Technology Has Revolutionized All Aspects of Biology 154
Restriction enzymes and DNA ligase are key tools in forming recombinant DNA molecules 154
Plasmids and lambda phage are choice vectors for DNA cloning in bacteria 155
Bacterial and yeast artificial chromosomes 157
Specific genes can be cloned from digests of genomic DNA 157
Complementary DNA prepared from mRNA can be expressed in host cells 160
Proteins with new functions can be created through directed changes in DNA 162
Recombinant methods enable the exploration of the functional effects of disease-causing mutations 163
5.3 Complete Genomes Have Been Sequenced and Analyzed 163
The genomes of organisms ranging from bacteria to multicellular eukaryotes have been sequenced 164
The sequencing of the human genome has been finished 165
Next-generation sequencing methods enable the rapid determination of a whole genome sequence 166
Comparative genomics has become a powerful research tool 166
5.4 Eukaryotic Genes Can Be Quantitated and Manipulated with Considerable Precision 167
Gene-expression levels can be comprehensively examined 167
New genes inserted into eukaryotic cells can be efficiently expressed 169
Transgenic animals harbor and express genes introduced into their germ lines 170
Gene disruption provides clues to gene function 170
RNA interference provides an additional tool for disrupting gene expression 171
Tumor-inducing plasmids can be used to introduce new genes into plant cells 172
Human gene therapy holds great promise for medicine 173
Chapter 6 Exploring Evolution and Bioinformatics 181
6.1 Homologs Are Descended from a Common Ancestor 182
6.2 Statistical Analysis of Sequence Alignments Can Detect Homology 183
The statistical significance of alignments can be estimated by shuffling 185
Distant evolutionary relationships can be detected through the use of substitution matrices 186
Databases can be searched to identify homologous sequences 189
6.3 Examination of Three-Dimensional Structure Enhances Our Understanding of Evolutionary Relationships 190
Tertiary structure is more conserved than primary structure 191
Knowledge of three-dimensional structures can aid in the evaluation of sequence alignments 192
Repeated motifs can be detected by aligning sequences with themselves 192
Convergent evolution illustrates common solutions to biochemical challenges 193
Comparison of RNA sequences can be a source of insight into RNA secondary structures 194
6.4 Evolutionary Trees Can Be Constructed on the Basis of Sequence Information 195
6.5 Modern Techniques Make the Experimental Exploration of Evolution Possible 196
Ancient DNA can sometimes be amplified and sequenced 196
Molecular evolution can be examined experimentally 197
Chapter 7 Hemoglobin:Portrait of a Protein in Action 203
7.1 Myoglobin and Hemoglobin Bind Oxygen at Iron Atoms in Heme 204
Changes in heme electronic structure upon oxygen binding are the basis for functional imaging studies 205
The structure of myoglobin prevents the release of reactive oxygen species 206
Human hemoglobin is an assembly of four myoglobin-like subunits 207
7.2 Hemoglobin Binds Oxygen Cooperatively 207
Oxygen binding markedly changes the quaternary structure of hemoglobin 209
Hemoglobin cooperativity can be potentially explained by several models 210
Structural changes at the heme groups are transmitted to the α1β1-α2β2 interface 212
2,3-Bisphosphoglycerate in red cells is crucial in determining the oxygen affinity of hemoglobin 212
Carbon monoxide can disrupt oxygen transport by hemoglobin 213
7.3 Hydrogen Ions and Carbon Dioxide Promote the Release of Oxygen:The Bohr Effect 214
7.4 Mutations in Genes Encoding Hemoglobin Subunits Can Result in Disease 216
Sickle-cell anemia results from the aggregation of mutated deoxyhemoglobin molecules 217
Thalassemia is caused by an imbalanced production of hemoglobin chains 218
The accumulation of free alpha-hemoglobin chains is prevented 219
Additional globins are encoded in the human genome 219
APPENDIX:Binding Models Can Be Formulated in Quantitative Terms:the Hill Plot and the Concerted Model 221
Chapter 8 Enzymes:Basic Concepts and Kinetics 227
8.1 Enzymes Are Powerful and Highly Specific Catalysts 228
Many enzymes require cofactors for activity 229
Enzymes can transform energy from one form into another 229
8.2 Free Energy Is a Useful Thermodynamic Function for Understanding Enzymes 230
The free-energy change provides information about the spontaneity but not the rate of a reaction 230
The standard free-energy change of a reaction is related to the equilibrium constant 231
Enzymes alter only the reaction rate and not the reaction equilibrium 232
8.3 Enzymes Accelerate Reactions by Facilitating the Formation of the Transition State 233
The formation of an enzyme-substrate complex is the first step in enzymatic catalysis 234
The active sites of enzymes have some common features 235
The binding energy between enzyme and substrate is important for catalysis 237
8.4 The Michaelis-Menten Equation Describes the Kinetic Properties of Many Enzymes 237
Kinetics is the study of reaction rates 237
The steady-state assumption facilitates a description of enzyme kinetics 238
Variations in KM can have physiological consequences 240
KM and Vmax values can be determined by several means 240
KM and Vmax values are important enzyme characteristics 241
kcat/KM is a measure of catalytic efficiency 242
Most biochemical reactions include multiple substrates 243
Allosteric enzymes do not obey Michaelis-Menten kinetics 245
8.5 Enzymes Can Be Inhibited by Specific Molecules 246
Reversible inhibitors are kinetically distinguishable 247
Irreversible inhibitors can be used to map the active site 249
Transition-state analogs are potent inhibitors of enzymes 251
Catalytic antibodies demonstrate the importance of selective binding of the transition state to enzymatic activity 251
Penicillin irreversibly inactivates a key enzyme in bacterial cell-wall synthesis 252
8.6 Enzymes Can Be Studied One Molecule at a Time 254
APPENDIX:Enzymes are Classified on the Basis of the Types of Reactions That They Catalyze 256
Chapter 9 Catalytic Strategies 261
A few basic catalytic principles are used by many enzymes 262
9.1 Proteases Facilitate a Fundamentally Difficult Reaction 263
Chymotrypsin possesses a highly reactive serine residue 263
Chymotrypsin action proceeds in two steps linked by a covalently bound intermediate 264
Serine is part of a catalytic triad that also includes histidine and aspartate 265
Catalytic triads are found in other hydrolytic enzymes 268
The catalytic triad has been dissected by site-directed mutagenesis 270
Cysteine,aspartyl,and metalloproteases are other major classes of peptide-cleaving enzymes 271
Protease inhibitors are important drugs 272
9.2 Carbonic Anhydrases Make a Fast Reaction Faster 274
Carbonic anhydrase contains a bound zinc ion essential for catalytic activity 275
Catalysis entails zinc activation of a water molecule 276
A proton shuttle facilitates rapid regeneration of the active form of the enzyme 277
Convergent evolution has generated zinc-based active sites in different carbonic anhydrases 279
9.3 Restriction Enzymes Catalyze Highly Specific DNA-Cleavage Reactions 279
Cleavage is by in-line displacement of 3’-oxygen from phosphorus by magnesium-activated water 280
Restriction enzymes require magnesium for catalytic activity 282
The complete catalytic apparatus is assembled only within complexes of cognate DNA molecules,ensuring specificity 283
Host-cell DNA is protected by the addition of methyl groups to specific bases 285
Type Ⅱ restriction enzymes have a catalytic core in common and are probably related by horizontal gene transfer 286
9.4 Myosins Harness Changes in Enzyme Conformation to Couple ATP Hydrolysis to Mechanical Work 287
ATP hydrolysis proceeds by the attack of water on the gamma-phosphoryl group 287
Formation of the transition state for ATP hydrolysis is associated with a substantial conformational change 288
The altered conformation of myosin persists for a substantial period of time 290
Myosins are a family of enzymes containing P-loop structures 291
Chapter 10 Regulatory Strategies 299
10.1 Aspartate Transcarbamoylase Is Allosterically Inhibited by the End Product of Its Pathway 300
Allosterically regulated enzymes do not follow Michaelis-Menten kinetics 301
ATCase consists of separable catalytic and regulatory subunits 301
Allosteric interactions in ATCase are mediated by large changes in quaternary structure 302
Allosteric regulators modulate the T-to-R equilibrium 305
10.2 Isozymes Provide a Means of Regulation Specific to Distinct Tissues and Developmental Stages 306
10.3 Covalent Modification Is a Means of Regulating Enzyme Activity 307
Kinases and phosphatases control the extent of protein phosphorylation 308
Phosphorylation is a highly effective means of regulating the activities of target proteins 310
Cyclic AMP activates protein kinase A by altering the quaternary structure 311
ATP and the target protein bind to a deep cleft in the catalytic subunit of protein kinase A 312
10.4 Many Enzymes Are Activated by Specific Proteolytic Cleavage 312
Chymotrypsinogen is activated by specific cleavage of a single peptide bond 313
Proteolytic activation of chymotrypsinogen leads to the formation of a substrate-binding site 314
The generation of trypsin from trypsinogen leads to the activation of other zymogens 315
Some proteolytic enzymes have specific inhibitors 316
Blood clotting is accomplished by a cascade of zymogen activations 317
Fibrinogen is converted by thrombin into a fibrin clot 318
Prothrombin is readied for activation by a vitamin K-dependent modification 320
Hemophilia revealed an early step in clotting 321
The clotting process must be precisely regulated 321
Chapter 11 Carbohydrates 329
11.1 Monosaccharides Are the Simplest Carbohydrates 330
Many common sugars exist in cyclic forms 332
Pyranose and furanose rings can assume different conformations 334
Glucose is a reducing sugar 335
Monosaccharides are joined to alcohols and amines through glycosidic bonds 336
Phosphorylated sugars are key intermediates in energy generation and biosyntheses 336
11.2 Monosaccharides Are Linked to Form Complex Carbohydrates 337
Sucrose,lactose,and maltose are the common disaccharides 337
Glycogen and starch are storage forms of glucose 338
Cellulose,a structural component of plants,is made of chains of glucose 338
11.3 Carbohydrates Can Be Linked to Proteins to Form Glycoproteins 339
Carbohydrates can be linked to proteins through asparagine(N-linked)or through serine or threonine(O-linked)residues 340
The glycoprotein erythropoietin is a vital hormone 340
Proteoglycans,composed of polysaccharides and protein,have important structural roles 341
Proteoglycans are important components of cartilage 342
Mucins are glycoprotein components of mucus 343
Protein glycosylation takes place in the lumen of the endoplasmic reticulum and in the Golgi complex 343
Specific enzymes are responsible for oligosaccharide assembly 345
Blood groups are based on protein glycosylation patterns 345
Errors in glycosylation can result in pathological conditions 346
Oligosaccharides can be “sequenced” 346
11.4 Lectins Are Specific Carbohydrate-Binding Proteins 347
Lectins promote interactions between cells 348
Lectins are organized into different classes 348
Influenza virus binds to sialic acid residues 349
Chapter 12 Lipids and Cell Membranes 357
Many common features underlie the diversity of biological membranes 358
12.1 Fatty Acids Are Key Constituents of Lipids 358
Fatty acid names are based on their parent hydrocarbons 358
Fatty acids vary in chain length and degree of unsaturation 359
12.2 There Are Three Common Types of Membrane Lipids 360
Phospholipids are the major class of membrane lipids 360
Membrane lipids can include carbohydrate moieties 361
Cholesterol is a lipid based on a steroid nucleus 362
Archaeal membranes are built from ether lipids with branched chains 362
A membrane lipid is an amphipathic molecule containing a hydrophilic and a hydrophobic moiety 363
12.3 Phospholipids and Glycolipids Readily Form Bimolecular Sheets in Aqueous Media 364
Lipid vesicles can be formed from phospholipids 365
Lipid bilayers are highly impermeable to ions and most polar molecules 366
12.4 Proteins Carry Out Most Membrane Processes 367
Proteins associate with the lipid bilayer in a variety of ways 367
Proteins interact with membranes in a variety of ways 368
Some proteins associate with membranes through covalently attached hydrophobic groups 371
Transmembrane helices can be accurately predicted from amino acid sequences 371
12.5 Lipids and Many Membrane Proteins Diffuse Rapidly in the Plane of the Membrane 373
The fluid mosaic model allows lateral movement but not rotation through the membrane 374
Membrane fluidity is controlled by fatty acid composition and cholesterol content 374
Lipid rafts are highly dynamic complexes formed between cholesterol and specific lipids 375
All biological membranes are asymmetric 375
12.6 Eukaryotic Cells Contain Compartments Bounded by Internal Membranes 376
Chapter 13 Membrane Channels and Pumps 383
The expression of transporters largely defines the metabolic activities of a given cell type 384
13.1 The Transport of Molecules Across a Membrane May Be Active or Passive 384
Many molecules require protein transporters to cross membranes 384
Free energy stored in concentration gradients can be quantified 385
13.2 Two Families of Membrane Proteins Use ATP Hydrolysis to Pump Ions and Molecules Across Membranes 386
P-type ATPases couple phosphorylation and conformational changes to pump calcium ions across membranes 386
Digitalis specifically inhibits the Na+-K+pump by blocking its dephosphorylation 389
P-type ATPases are evolutionarily conserved and play a wide range of roles 390
Multidrug resistance highlights a family of membrane pumps with ATP-binding cassette domains 390
13.3 Lactose Permease Is an Archetype of Secondary Transporters That Use One Concentration Gradient to Power the Formation of Another 392
13.4 Specific Channels Can Rapidly Transport Ions Across Membranes 394
Action potentials are mediated by transient changes in Na+and K+permeability 394
Patch-clamp conductance measurements reveal the activities of single channels 395
The structure of a potassium ion channel is an archetype for many ion-channel structures 395
The structure of the potassium ion channel reveals the basis of ion specificity 396
The structure of the potassium ion channel explains its rapid rate of transport 399
Voltage gating requires substantial conformational changes in specific ion-channel domains 399
A channel can be activated by occlusion of the pore:the ball-and-chain model 400
The acetylcholine receptor is an archetype for ligand-gated ion channels 401
Action potentials integrate the activities of several ion channels working in concert 402
Disruption of ion channels by mutations or chemicals can be potentially life threatening 404
13.5 Gap Junctions Allow Ions and Small Molecules to Flow Between Communicating Cells 405
13.6 Specific Channels Increase the Permeability of Some Membranes to Water 406
Chapter 14 Signal-Transduction Pathways 415
Signal transduction depends on molecular circuits 416
14.1 Heterotrimeric G Proteins Transmit Signals and Reset Themselves 417
Ligand binding to 7TM receptors leads to the activation of heterotrimeric G proteins 419
Activated G proteins transmit signals by binding to other proteins 420
Cyclic AMP stimulates the phosphorylation of many target proteins by activating protein kinase A 420
G proteins spontaneously reset themselves through GTP hydrolysis 421
Some 7TM receptors activate the phosphoinositide cascade 422
Calcium ion is a widely used second messenger 423
Calcium ion often activates the regulatory protein calmodulin 424
14.2 Insulin Signaling:Phosphorylation Cascades Are Central to Many Signal-Transduction Processes 425
The insulin receptor is a dimer that closes around a bound insulin molecule 426
Insulin binding results in the cross-phosphorylation and activation of the insulin receptor 426
The activated insulin-receptor kinase initiates a kinase cascade 426
Insulin signaling is terminated by the action of phosphatases 429
14.3 EGF Signaling:Signal-Transduction Pathways Are Poised to Respond 429
EGF binding results in the dimerization of the eGF receptor 429
The EGF receptor undergoes phosphorylation of its carboxyl-terminal tail 431
EGF signaling leads to the activation of Ras,a small G protein 431
Activated Ras initiates a protein kinase cascade 432
EGF signaling is terminated by protein phosphatases and the intrinsic GTPase activity of Ras 432
14.4 Many Elements Recur with Variation in Different Signal-Transduction Pathways 433
14.5 Defects in Signal-Transduction Pathways Can Lead to Cancer and Other Diseases 434
Monoclonal antibodies can be used to inhibit signal-transduction pathways activated in tumors 434
Protein kinase inhibitors can be effective anticancer drugs 435
Cholera and whooping cough are due to altered G-protein activity 435
Part Ⅱ TRANSDUCING AND STORING ENERGY 443
Chapter 15 Metabolism:Basic Concepts and Design 443
15.1 Metabolism Is Composed of Many Coupled,Interconnecting Reactions 444
Metabolism consists of energy-yielding and energy-requiring reactions 444
A thermodynamically unfavorable reaction can be driven by a favorable reaction 445
15.2 ATP Is the Universal Currency of Free Energy in Biological Systems 446
ATP hydrolysis is exergonic 446
ATP hydrolysis drives metabolism by shifting the equilibrium of coupled reactions 447
The high phosphoryl potential of ATP results from structural differences between ATP and its hydrolysis products 449
Phosphoryl-transfer potential is an important form of cellular energy transformation 450
15.3 The Oxidation of Carbon Fuels Is an Important Source of Cellular Energy 451
Compounds with high phosphoryl-transfer potential can couple carbon oxidation to ATP synthesis 452
Ion gradients across membranes provide an important form of cellular energy that can be coupled to ATP synthesis 453
Energy from foodstuffs is extracted in three stages 453
15.4 Metabolic Pathways Contain Many Recurring Motifs 454
Activated carriers exemplify the modular design and economy of metabolism 454
Many activated carriers are derived from vitamins 457
Key reactions are reiterated throughout metabolism 459
Metabolic processes are regulated in three principal ways 461
Aspects of metabolism may have evolved from an RNA world 463
Chapter 16 Glycolysis and Gluconeogenesis 469
Glucose is generated from dietary carbohydrates 470
Glucose is an important fuel for most organisms 471
16.1 Glycolysis Is an Energy-Conversion Pathway in Many Organisms 471
Hexokinase traps glucose in the cell and begins glycolysis 471
Fructose 1,6-bisphosphate is generated from glucose 6-phosphate 473
The six-carbon sugar is cleaved into two three-carbon fragments 474
Mechanism:Triose phosphate isomerase salvages a three-carbon fragment 475
The oxidation of an aldehyde to an acid powers the formation of a compound with high phosphoryl-transfer potential 476
Mechanism:Phosphorylation is coupled to the oxidation of glyceraldehyde 3-phosphate by a thioester intermediate 478
ATP is formed by phosphoryl transfer from 1,3-bisphosphoglycerate 479
Additional ATP is generated with the formation of pyruvate 480
Two ATP molecules are formed in the conversion of glucose into pyruvate 481
NAD+is regenerated from the metabolism of pyruvate 482
Fermentations provide usable energy in the absence of oxygen 484
The binding site for NAD+is similar in many dehydrogenases 485
Fructose and galactose are converted into glycolytic intermediates 485
Many adults are intolerant of milk because they are deficient in lactase 487
Galactose is highly toxic if the transferase is missing 488
16.2 The Glycolytic Pathway Is Tightly Controlled 488
Glycolysis in muscle is regulated to meet the need for ATP 489
The regulation of glycolysis in the liver illustrates the biochemical versatility of the liver 490
A family of transporters enables glucose to enter and leave animal cells 493
Cancer and exercise training affect glycolysis in a similar fashion 494
16.3 Glucose Can Be Synthesized from Noncarbohydrate Precursors 495
Gluconeogenesis is not a reversal of glycolysis 497
The conversion of pyruvate into phosphoenolpyruvate begins with the formation of oxaloacetate 498
Oxaloacetate is shuttled into the cytoplasm and converted into phosphoenolpyruvate 499
The conversion of fructose 1,6-bisphosphate into fructose 6-phosphate and orthophosphate is an irreversible step 500
The generation of free glucose is an important control point 500
Six high-transfer-potential phosphoryl groups are spent in synthesizing glucose from pyruvate 501
16.4 Gluconeogenesis and Glycolysis Are Reciprocally Regulated 502
Energy charge determines whether glycolysis or gluconeogenesis will be most active 502
The balance between glycolysis and gluconeogenesis in the liver is sensitive to blood-glucose concentration 503
Substrate cycles amplify metabolic signals and produce heat 505
Lactate and alanine formed by contracting muscle are used by other organs 505
Glycolysis and gluconeogenesis are evolutionarily intertwined 507
Chapter 17 The Citric Acid Cycle 515
The citric acid cycle harvests high-energy electrons 516
17.1 Pyruvate Dehydrogenase Links Glycolysis to the Citric Acid Cycle 517
Mechanism:The synthesis of acetyl coenzyme a frompyruvate requires three enzymes and five coenzymes 518
Flexible linkages allow lipoamide to move between different active sites 520
17.2 The Citric Acid Cycle Oxidizes Two-Carbon Units 521
Citrate synthase forms citrate from oxaloacetate and acetyl coenzyme A 522
Mechanism:The mechanism of citrate synthase prevents undesirable reactions 522
Citrate is isomerized into isocitrate 524
Isocitrate is oxidized and decarboxylated to alpha-ketoglutarate 524
Succinyl coenzyme A is formed by the oxidative decarboxylation of alpha-ketoglutarate 525
A compound with high phosphoryl-transfer potential is generated from succinyl coenzyme A 525
Mechanism:Succinyl coenzyme A synthetase transforms types of biochemical energy 526
Oxaloacetate is regenerated by the oxidation of succinate 527
The citric acid cycle produces high-transfer-potential electrons,ATP,and CO2 528
17.3 Entry to the Citric Acid Cycle and Metabolism Through It Are Controlled 530
The pyruvate dehydrogenase complex is regulated allosterically and by reversible phosphorylation 531
The citric acid cycle is controlled at several points 532
Defects in the citric acid cycle contribute to the development of cancer 533
17.4 The Citric Acid Cycle Is a Source of Biosynthetic Precursors 534
The citric acid cycle must be capable of being rapidly replenished 534
The disruption of pyruvate metabolism is the cause of beriberi and poisoning by mercury and arsenic 535
The citric acid cycle may have evolved from preexisting pathways 536
17.5 The Glyoxylate Cycle Enables Plants and Bacteria to Grow on Acetate 536
Chapter 18 Oxidative Phosphorylation 543
18.1 Eukaryotic Oxidative Phosphorylation Takes Place in Mitochondria 544
Mitochondria are bounded by a double membrane 544
Mitochondria are the result of an endosymbiotic event 545
18.2 Oxidative Phosphorylation Depends on Electron Transfer 546
The electron-transfer potential of an electron is measured as redox potential 546
A 1.14-volt potential difference between NADH and molecular oxygen drives electron transport through the chain and favors the formation of a proton gradient 548
18.3 The Respiratory Chain Consists of Four Complexes:Three Proton Pumps and a Physical Link to the Citric Acid Cycle 549
The high-potential electrons of NADH enter the respiratory chain at NADH-Qoxidoreductase 551
Ubiquinol is the entry point for electrons from FADH2 of flavoproteins 553
Electrons flow from ubiquinol to cytochrome c through Q-cytochrome c oxidoreductase 553
The Qcycle funnels electrons from a two-electron carrier to a one-electron carrier and pumps protons 554
Cytochrome c oxidase catalyzes the reduction of molecular oxygen to water 555
Toxic derivatives of molecular oxygen such as superoxide radical are scavenged by protective enzymes 558
Electrons can be transferred between groups that are not in contact 560
The conformation of cytochrome c has remained essentially constant for more than a billion years 561
18.4 A Proton Gradient Powers the Synthesis of ATP 561
ATP synthase is composed of a proton-conducting unit and a catalytic unit 563
Proton flow through ATP synthase leads to the release of tightly bound ATP:The binding-change mechanism 564
Rotational catalysis is the world’s smallest molecular motor 565
Proton flow around the c ring powers ATP synthesis 566
ATP synthase and G proteins have several common features 568
18.5 Many Shuttles Allow Movement Across Mitochondrial Membranes 568
Electrons from cytoplasmic NADH enter mitochondria by shuttles 569
The entry of ADP into mitochondria is coupled to the exit of ATP by ATP-ADP translocase 570
Mitochondrial transporters for metabolites have a common tripartite structure 571
18.6 The Regulation of Cellular Respiration Is Governed Primarily by the Need for ATP 572
The complete oxidation of glucose yields about 30 molecules of ATP 572
The rate of oxidative phosphorylation is determined by the need for ATP 573
Regulated uncoupling leads to the generation of heat 574
Oxidative phosphorylation can be inhibited at many stages 576
Mitochondrial diseases are being discovered 576
Mitochondria play a key role in apoptosis 577
Power transmission by proton gradients is a central motif of bioenergetics 577
Chapter 19 The Light Reactions of Photosynthesis 585
Photosynthesis converts light energy into chemical energy 586
19.1 Photosynthesis Takes Place in Chloroplasts 587
The primary events of photosynthesis take place in thylakoid membranes 587
Chloroplasts arose from an endosymbiotic event 588
19.2 Light Absorption by Chlorophyll Induces Electron Transfer 588
A special pair of chlorophylls initiate charge separation 589
Cyclic electron flow reduces the cytochrome of the reaction center 592
19.3 Two Photosystems Generate a Proton Gradient and NADPH in Oxygenic Photosynthesis 592
Photosystem Ⅱ transfers electrons from water to plastoquinone and generates a proton gradient 592
Cytochrome bf links photosystem Ⅱ to photosystem Ⅰ 595
Photosystem I uses light energy to generate reduced ferredoxin,a powerful reductant 595
Ferredoxin-NADP+reductase converts NADP+into NADPH 596
19.4 A Proton Gradient Across the Thylakoid Membrane Drives ATP Synthesis 597
The ATP synthase of chloroplasts closely resembles those of mitochondria and prokaryotes 598
Cyclic electron flow through photosystem I leads to the production of ATP instead of NADPH 599
The absorption of eight photons yields one O2,two NADPH,and three ATP molecules 600
19.5 Accessory Pigments Funnel Energy into Reaction Centers 601
Resonance energy transfer allows energy to move from the site of initial absorbance to the reaction center 601
Light-harvesting complexes contain additional chlorophylls and carotinoids 602
The components of photosynthesis are highly organized 603
Many herbicides inhibit the light reactions of photosynthesis 604
19.6 The Ability to Convert Light into Chemical Energy Is Ancient 604
Chapter 20 The Calvin Cycle and Pentose Phosphate Pathway 609
20.1 The Calvin Cycle Synthesizes Hexoses from Carbon Dioxide and Water 610
Carbon dioxide reacts with ribulose 1,5-bisphosphate to form two molecules of 3-phosphoglycerate 611
Rubisco activity depends on magnesium and carbamate 612
Rubisco also catalyzes a wasteful oxygenase reaction:Catalytic imperfection 613
Hexose phosphates are made from phosphoglycerate,and ribulose 1,5-bisphosphate is regenerated 614
Three ATP and two NADPH molecules are used to bring carbon dioxide to the level of a hexose 617
Starch and sucrose are the major carbohydrate stores in plants 617
20.2 The Activity of the Calvin Cycle Depends on Environmental Conditions 617
Rubisco is activated by light-driven changes in proton and magnesium ion concentrations 618
Thioredoxin plays a key role in regulating the Calvin cycle 618
The C4 pathway of tropical plants accelerates photosynthesis by concentrating carbon dioxide 619
Crassulacean acid metabolism permits growth in arid ecosystems 620
20.3 The Pentose Phosphate Pathway Generates NADPH and Synthesizes Five-Carbon Sugars 621
Two molecules of NADPH are generated in the conversion of glucose 6-phosphate into ribulose 5-phosphate 621
The pentose phosphate pathway and glycolysis are linked by transketolase and transaldolase 621
Mechanism:Transketolase and transaldolase stabilize carbanionic intermediates by different mechanisms 624
20.4 The Metabolism of Glucose 6-phosphate by the Pentose Phosphate Pathway Is Coordinated with Glycolysis 626
The rate of the pentose phosphate pathway is controlled by the level of NADP+ 626
The flow of glucose 6-phosphate depends on the need for NADPH,ribose 5-phosphate,and ATP 627
Through the looking-glass:The Calvin cycle and the pentose phosphate pathway are mirror images 629
20.5 Glucose 6-phosphate Dehydrogenase Plays a Key Role in Protection Against Reactive Oxygen Species 629
Glucose 6-phosphate dehydrogenase deficiency causes a drug-induced hemolytic anemia 629
A deficiency of glucose 6-phosphate dehydrogenase confers an evolutionary advantage in some circumstances 631
Chapter 21 Glycogen Metabolism 637
Glycogen metabolism is the regulated release and storage of glucose 638
21.1 Glycogen Breakdown Requires the Interplay of Several Enzymes 639
Phosphorylase catalyzes the phosphorolytic cleavage of glycogen to release glucose 1-phosphate 639
Mechanism:Pyridoxal phosphate participates in the phosphorolytic cleavage of glycogen 640
A debranching enzyme also is needed for the breakdown of glycogen 641
Phosphoglucomutase converts glucose 1-phosphate into glucose 6-phosphate 642
The liver contains glucose 6-phosphatase,a hydrolytic enzyme absent from muscle 643
21.2 Phosphorylase Is Regulated by Allosteric Interactions and Reversible Phosphorylation 643
Muscle phosphorylase is regulated by the intracellular energy charge 643
Liver phosphorylase produces glucose for use by other tissues 645
Phosphorylase kinase is activated by phosphorylation and calcium ions 645
21.3 Epinephrine and Glucagon Signal the Need for Glycogen Breakdown 646
G proteins transmit the signal for the initiation of glycogen breakdown 646
Glycogen breakdown must be rapidly turned off when necessary 648
The regulation of glycogen phosphorylase became more sophisticated as the enzyme evolved 649
21.4 Glycogen Is Synthesized and Degraded by Different Pathways 649
UDP-glucose is an activated form of glucose 649
Glycogen synthase catalyzes the transfer of glucose from UDP-glucose to a growing chain 650
A branching enzyme forms α-1,6 linkages 651
Glycogen synthase is the key regulatory enzyme in glycogen synthesis 651
Glycogen is an efficient storage form of glucose 651
21.5 Glycogen Breakdown and Synthesis Are Reciprocally Regulated 652
Protein phosphatase 1 reverses the regulatory effects of kinases on glycogen metabolism 653
Insulin stimulates glycogen synthesis by inactivating glycogen synthase kinase 654
Glycogen metabolism in the liver regulates the blood-glucose level 655
A biochemical understanding of glycogen-storage diseases is possible 656
Chapter 22 Fatty Acid Metabolism 663
Fatty acid degradation and synthesis mirror each other in their chemical reactions 664
22.1 Triacylglycerols Are Highly Concentrated Energy Stores 665
Dietary lipids are digested by pancreatic lipases 665
Dietary lipids are transported in chylomicrons 666
22.2 The Use of Fatty Acids As Fuel Requires Three Stages of Processing 667
Triacylglycerols are hydrolyzed by hormone-stimulated lipases 667
Fatty acids are linked to coenzyme A before they are oxidized 668
Carnitine carries long-chain activated fatty acids into the mitochondrial matrix 669
Acetyl CoA,NADH,and FADH2 are generated in each round of fatty acid oxidation 670
The complete oxidation of palmitate yields 106 molecules of ATP 671
22.3 Unsaturated and Odd-Chain Fatty Acids Require Additional Steps for Degradation 672
An isomerase and a reductase are required for the oxidation of unsaturated fatty acids 672
Odd-chain fatty acids yield propionyl CoA in the final thiolysis step 673
Vitamin B12 contains a corrin ring and a cobalt atom 674
Mechanism:Methylmalonyl CoA mutase catalyzes a rearrangement to form succinyl CoA 675
Fatty acids are also oxidized in peroxisomes 676
Ketone bodies are formed from acetyl CoA when fat breakdown predominates 677
Ketone bodies are a major fuel in some tissues 678
Animals cannot convert fatty acids into glucose 680
22.4 Fatty Acids Are Synthesized by Fatty Acid Synthase 680
Fatty acids are synthesized and degraded by different pathways 680
The formation of malonyl CoA is the committed step in fatty acid synthesis 681
Intermediates in fatty acid synthesis are attached to an acyl carrier protein 681
Fatty acid synthesis consists of a series of condensation,reduction,dehydration,and reduction reactions 682
Fatty acids are synthesized by a multifunctional enzyme complex in animals 683
The synthesis of palmitate requires 8 molecules of acetyl CoA,14 molecules of NADPH,and 7 molecules of ATP 685
Citrate carries acetyl groups from mitochondria to the cytoplasm for fatty acid synthesis 686
Several sources supply NADPH for fatty acid synthesis 686
Fatty acid synthase inhibitors may be useful drugs 687
22.5 The Elongation and Unsaturation of Fatty Acids Are Accomplished by Accessory Enzyme Systems 687
Membrane-bound enzymes generate unsaturated fatty acids 688
Eicosanoid hormones are derived from polyunsaturated fatty acids 688
22.6 Acetyl CoA Carboxylase Plays a Key Role in Controlling Fatty Acid Metabolism 690
Acetyl CoA carboxylase is regulated by conditions in the cell 690
Acetyl CoA carboxylase is regulated by a variety of hormones 690
Chapter 23 Protein Turnover and Amino Acid Catabolism 697
23.1 Proteins Are Degraded to Amino Acids 698
The digestion of dietary proteins begins in the stomach and is completed in the intestine 698
Cellular proteins are degraded at different rates 699
23.2 Protein Turnover Is Tightly Regulated 699
Ubiquitin tags proteins for destruction 699
The proteasome digests the ubiquitin-tagged proteins 701
The ubiquitin pathway and the proteasome have prokaryotic counterparts 701
Protein degradation can be used to regulate biological function 702
23.3 The First Step in Amino Acid Degradation Is the Removal of Nitrogen 704
Alpha-amino groups are converted into ammonium ions by the oxidative deamination of glutamate 704
Mechanism:Pyridoxal phosphate forms Schiff-base intermediates in aminotransferases 705
Aspartate aminotransferase is an archetypal pyridoxal-dependent transaminase 706
Pyridoxal phosphate enzymes catalyze a wide array of reactions 707
Serine and threonine can be directly deaminated 708
Peripheral tissues transport nitrogen to the liver 708
23.4 Ammonium Ion Is Converted into Urea in Most Terrestrial Vertebrates 709
The urea cycle begins with the formation of carbamoyl phosphate 709
The urea cycle is linked to gluconeogenesis 711
Urea-cycle enzymes are evolutionarily related to enzymes in other metabolic pathways 712
Inherited defects of the urea cycle cause hyperammonemia and can lead to brain damage 712
Urea is not the only means of disposing of excess nitrogen 713
23.5 Carbon Atoms of Degraded Amino Acids Emerge As Major Metabolic Intermediates 714
Pyruvate is an entry point into metabolism for a number of amino acids 715
Oxaloacetate is an entry point into metabolism for aspartate and asparagine 716
Alpha-ketoglutarate is an entry point into metabolism for five-carbon amino acids 716
Succinyl coenzyme A is a point of entry for several nonpolar amino acids 717
Methionine degradation requires the formation of a key methyl donor,S-adenosylmethionine 717
The branched-chain amino acids yield acetyl CoA,acetoacetate,or propionyl CoA 717
Oxygenases are required for the degradation of aromatic amino acids 719
23.6 Inborn Errors of Metabolism Can Disrupt Amino Acid Degradation 721
Part Ⅲ SYNTHESIZING THE MOLECULES OF LIFE 729
Chapter 24 The Biosynthesis of Amino Acids 729
Amino acid synthesis requires solutions to three key biochemical problems 730
24.1 Nitrogen Fixation:Microorganisms Use ATP and a Powerful Reductant to Reduce Atmospheric Nitrogen to Ammonia 730
The iron-molybdenum cofactor of nitrogenase binds and reduces atmospheric nitrogen 731
Ammonium ion is assimilated into an amino acid through glutamate and glutamine 733
24.2 Amino Acids Are Made from Intermediates of the Citric Acid Cycle and Other Major Pathways 735
Human beings can synthesize some amino acids but must obtain others from the diet 735
Aspartate,alanine,and glutamate are formed by the addition of an amino group to an alpha-ketoacid 736
A common step determines the chirality of all amino acids 737
The formation of asparagine from aspartate requires an adenylated intermediate 737
Glutamate is the precursor of glutamine,proline,and arginine 738
3-Phosphoglycerate is the precursor of serine,cysteine,and glycine 738
Tetrahydrofolate carries activated one-carbon units at several oxidation levels 739
S-Adenosylmethionine is the major donor of methyl groups 740
Cysteine is synthesized from serine and homocysteine 742
High homocysteine levels correlate with vascular disease 743
Shikimate and chorismate are intermediates in the biosynthesis of aromatic amino acids 743
Tryptophan synthase illustrates substrate channeling in enzymatic catalysis 746
24.3 Feedback Inhibition Regulates Amino Acid Biosynthesis 747
Branched pathways require sophisticated regulation 747
An enzymatic cascade modulates the activity of glutamine synthetase 749
24.4 Amino Acids Are Precursors of Many Biomolecules 750
Glutathione,a gamma-glutamyl peptide,serves as a sulfhydryl buffer and an antioxidant 751
Nitric oxide,a short-lived signal molecule,is formed from arginine 751
Porphyrins are synthesized from glycine and succinyl coenzyme A 752
Porphyrins accumulate in some inherited disorders of porphyrin metabolism 754
Chapter 25 Nucleotide Biosynthesis 761
Nucleotides can be synthesized by de novo or salvage pathways 762
25.1 The Pyrimidine Ring Is Assembled de Novo or Recovered by Salvage Pathways 763
Bicarbonate and other oxygenated carbon compounds are activated by phosphorylation 763
The side chain of glutamine can be hydrolyzed to generate ammonia 763
Intermediates can move between active sites by channeling 763
Orotate acquires a ribose ring from PRPP to form a pyrimidine nucleotide and is converted into uridylate 764
Nucleotide mono-,di-,and triphosphates are interconvertible 765
CTP is formed by amination of UTP 765
Salvage pathways recycle pyrimidine bases 766
25.2 Purine Bases Can Be Synthesized de Novo or Recycled by Salvage Pathways 766
The purine ring system is assembled on ribose phosphate 766
The purine ring is assembled by successive steps of activation by phosphorylation followed by displacement 767
AMP and GMP are formed from IMP 769
Enzymes of the purine synthesis pathway associate with one another in vivo 770
Salvage pathways economize intracellular energy expenditure 770
25.3 Deoxyribonucleotides Are Synthesized by the Reduction of Ribonucleotides Through a Radical Mechanism 771
Mechanism:A tyrosyl radical is critical to the action of ribonucleotide reductase 771
Stable radicals other than tyrosyl radical are employed by other ribonucleotide reductases 773
Thymidylate is formed by the methylation of deoxyuridylate 774
Dihydrofolate reductase catalyzes the regeneration of tetrahydrofolate,a one-carbon carrier 775
Several valuable anticancer drugs block the synthesis of thymidylate 775
25.4 Key Steps in Nucleotide Biosynthesis Are Regulated by Feedback Inhibition 776
Pyrimidine biosynthesis is regulated by aspartate transcarbamoylase 777
The synthesis of purine nucleotides is controlled by feedback inhibition at several sites 777
The synthesis of deoxyribonucleotides is controlled by the regulation of ribonucleotide reductase 778
25.5 Disruptions in Nucleotide Metabolism Can Cause Pathological Conditions 778
The loss of adenosine deaminase activity results in severe combined immunodeficiency 778
Gout is induced by high serum levels of urate 779
Lesch-Nyhan syndrome is a dramatic consequence of mutations in a salvage-pathway enzyme 780
Folic acid deficiency promotes birth defects such as spina bifida 781
Chapter 26 The Biosynthesis of Membrane Lipids and Steroids 787
26.1 Phosphatidate Is a Common Intermediate in the Synthesis of Phospholipids and Triacylglycerols 788
The synthesis of phospholipids requires an activated intermediate 789
Sphingolipids are synthesized from ceramide 791
Gangliosides are carbohydrate-rich sphingolipids that contain acidic sugars 792
Sphingolipids confer diversity on lipid structure and function 793
Respiratory distress syndrome and Tay-Sachs disease result from the disruption of lipid metabolism 793
Phosphatiditic acid phosphatase is a key regulatory enzyme in lipid metabolism 794
26.2 Cholesterol Is Synthesized from Acetyl Coenzyme A in Three Stages 795
The synthesis of mevalonate,which is activated as isopentenyl pyrophosphate,initiates the synthesis of cholesterol 795
Squalene(C30)is synthesized from six molecules of isopentenyl pyrophosphate(C5) 796
Squalene cyclizes to form cholesterol 797
26.3 The Complex Regulation of Cholesterol Biosynthesis Takes Place at Several Levels 798
Lipoproteins transport cholesterol and triacylglycerols throughout the organism 801
The blood levels of certain lipoproteins can serve diagnostic purposes 802
Low-density lipoproteins play a central role in cholesterol metabolism 803
The absence of the LDL receptor leads to hypercholesterolemia and atherosclerosis 804
Mutations in the LDL receptor prevent LDL release and result in receptor destruction 805
HDL appears to protect against arteriosclerosis 806
The clinical management of cholesterol levels can be understood at a biochemical level 807
26.4 Important Derivatives of Cholesterol Include Bile Salts and Steroid Hormones 807
Letters identify the steroid rings and numbers identify the carbon atoms 809
Steroids are hydroxylated by cytochrome P450 monooxygenases that use NADPH and O2 809
The cytochrome P450 system is widespread and performs a protective function 810
Pregnenolone,a precursor of many other steroids,is formed from cholesterol by cleavage of its side chain 811
Progesterone and corticosteroids are synthesized from pregnenolone 811
Androgens and estrogens are synthesized from pregnenolone 812
Vitamin D is derived from cholesterol by the ring-splitting activity of light 813
Chapter 27 The Integration of Metabolism 821
27.1 Caloric Homeostasis Is a Means of Regulating Body Weight 822
27.2 The Brain Plays a Key Role in Caloric Homeostasis 824
Signals from the gastrointestinal tract induce feelings of satiety 824
Leptin and insulin regulate long-term control over caloric homeostasis 825
Leptin is one of several hormones secreted by adipose tissue 826
Leptin resistance may be a contributing factor to obesity 827
Dieting is used to combat obesity 827
27.3 Diabetes Is a Common Metabolic Disease Often Resulting from Obesity 828
Insulin initiates a complex signal-transduction pathway in muscle 828
Metabolic syndrome often precedes type 2 diabetes 830
Excess fatty acids in muscle modify metabolism 830
Insulin resistance in muscle facilitates pancreatic failure 831
Metabolic derangements in type 1 diabetes result from insulin insufficiency and glucagon excess 832
27.4 Exercise Beneficially Alters the Biochemistry of Cells 833
Mitochondrial biogenesis is stimulated by muscular activity 834
Fuel choice during exercise is determined by the intensity and duration of activity 835
27.5 Food Intake and Starvation Induce Metabolic Changes 836
The starved-fed cycle is the physiological response to a fast 837
Metabolic adaptations in prolonged starvation minimize protein degradation 838
27.6 Ethanol Alters Energy Metabolism in the Liver 840
Ethanol metabolism leads to an excess of NADH 840
Excess ethanol consumption disrupts vitamin metabolism 842
Chapter 28 DNA Replication,Repair,and Recombination 849
28.1 DNA Replication Proceeds by the Polymerization of Deoxyribonucleoside Triphosphates Along a Template 850
DNA polymerases require a template and a primer 850
All DNA polymerases have structural features in common 851
Two bound metal ions participate in the polymerase reaction 851
The specificity of replication is dictated by complementarity of shape between bases 852
An RNA primer synthesized by primase enables DNA synthesis to begin 853
One strand of DNA is made continuously,whereas the other strand is synthesized in fragments 853
DNA ligase joins ends of DNA in duplex regions 854
The separation of DNA strands requires specific helicases and ATP hydrolysis 854
28.2 DNA Unwinding and Supercoiling Are Controlled by Topoisomerases 855
The linking number of DNA,a topological property,determines the degree of supercoiling 856
Topoisomerases prepare the double helix for unwinding 858
Type Ⅰ topoisomerases relax supercoiled structures 858
Type Ⅱ topoisomerases can introduce negative supercoils through coupling to ATP hydrolysis 859
28.3 DNA Replication Is Highly Coordinated 861
DNA replication requires highly processive polymerases 861
The leading and lagging strands are synthesized in a coordinated fashion 862
DNA replication in Escherichia coli begins at a unique site 864
DNA synthesis in eukaryotes is initiated at multiple sites 865
Telomeres are unique structures at the ends of linear chromosomes 866
Telomeres are replicated by telomerase,a specialized polymerase that carries its own RNA template 867
28.4 Many Types of DNA Damage Can Be Repaired 867
Eerrors can arise in DNA replication 867
Bases can be damaged by oxidizing agents,alkylating agents,and light 868
DNA damage can be detected and repaired by a variety of systems 869
The presence of thymine instead of uracil in DNA permits the repair of deaminated cytosine 871
Some genetic diseases are caused by the expansion of repeats of three nucleotides 872
Many cancers are caused by the defective repair of DNA 872
Many potential carcinogens can be detected by their mutagenic action on bacteria 873
28.5 DNA Recombination Plays Important Roles in Replication,Repair,and Other Processes 874
RecA can initiate recombination by promoting strand invasion 874
Some recombination reactions proceed through Holliday-junction intermediates 875
Chapter 29 RNA Synthesis and Processing 883
RNA synthesis comprises three stages:Initiation,elongation,and termination 884
29.1 RNA Polymerases Catalyze Transcription 885
RNA chains are formed de novo and grow in the 5’-to-3’ direction 886
RNA polymerases backtrack and correct errors 888
RNA polymerase binds to promoter sites on the DNA template to initiate transcription 888
Sigma subunits of RNA polymerase recognize promoter sites 889
RNA polymerases must unwind the template double helix for transcription to take place 890
Elongation takes place at transcription bubbles that move along the DNA template 890
Sequences within the newly transcribed RNA signal termination 891
Some messenger RNAs directly sense metabolite concentrations 892
The rho protein helps to terminate the transcription of some genes 892
Some antibiotics inhibit transcription 893
Precursors of transfer and ribosomal RNA are cleaved and chemically modified after transcription in prokaryotes 895
29.2 Transcription in Eukaryotes Is Highly Regulated 896
Three types of RNA polymerase synthesize RNA in eukaryotic cells 897
Three common elements can be found in the RNA polymerase Ⅱ promoter region 898
The TFIID protein complex initiates the assembly of the active transcription complex 899
Multiple transcription factors interact with eukaryotic promoters 900
Enhancer sequences can stimulate transcription at start sites thousands of bases away 900
29.3 The Transcription Products of Eukaryotic Polymerases Are Processed 901
RNA polymerase Ⅰ produces three ribosomal RNAs 901
RNA polymerase Ⅲ produces transfer RNA 902
The product of RNA polymerase Ⅱ,the pre-mRNA transcript,acquires a 5’ cap and a 3’ poly(A)tail 902
Small regulatory RNAs are cleaved from larger precursors 904
RNA editing changes the proteins encoded by mRNA 904
Sequences at the ends of introns specify splice sites in mRNA precursors 905
Splicing consists of two sequential transesterification reactions 906
Small nuclear RNAs in spliceosomes catalyze the splicing of mRNA precursors 907
Transcription and processing of mRNA are coupled 909
Mutations that affect pre-mRNA splicing cause disease 909
Most human pre-mRNAS can be spliced in alternative ways to yield different proteins 910
29.4 The Discovery of Catalytic RNA Was Revealing in Regard to Both Mechanism and Evolution 911
Chapter 30 Protein Synthesis 921
30.1 Protein Synthesis Requires the Translation of Nucleotide Sequences into Amino Acid Sequences 922
The synthesis of long proteins requires a low error frequency 922
Transfer RNA molecules have a common design 923
Some transfer RNA molecules recognize more than one codon because of wobble in base-pairing 925
30.2 Aminoacyl Transfer RNA Synthetases Read the Genetic Code 927
Amino acids are first activated by adenylation 927
Aminoacyl-tRNA synthetases have highly discriminating amino acid activation sites 928
Proofreading by aminoacyl-tRNA synthetases increases the fidelity of protein synthesis 929
Synthetases recognize various features of transfer RNA molecules 930
Aminoacyl-tRNA synthetases can be divided into two classes 931
30.3 The Ribosome Is the Site of Protein Synthesis 931
Ribosomal RNAs(5S,16S,and 23S rRNA)play a central role in protein synthesis 932
Ribosomes have three tRNA-binding sites that bridge the 30s and 50s subunits 934
The start signal is usually AUG preceded by several bases that pair with 16S rRNA 934
Bacterial protein synthesis is initiated by formylmethionyl transfer RNA 935
Formylmethionyl-tRNAf is placed in the P site of the ribosome in the formation of the 70S initiation complex 936
Elongation factors deliver aminoacyl-tRNA to the ribosome 936
Peptidyl transferase catalyzes peptide-bond synthesis 937
The formation of a peptide bond is followed by the GTP-driven translocation of tRNAs and mRNA 938
Protein synthesis is terminated by release factors that read stop codons 940
30.4 Eukaryotic Protein Synthesis Differs from Prokaryotic Protein Synthesis Primarily in Translation Initiation 941
Mutations in initiation factor 2 cause a curious pathological condition 942
30.5 A Variety of Antibiotics and Toxins Can Inhibit Protein Synthesis 943
Some antibiotics inhibit protein synthesis 943
Diphtheria toxin blocks protein synthesis in eukary
- 《立法的尊严=The Dignity of Legislation》(美)杰里米·沃尔德伦(Jeremy Waldron)著 2019
- 《变革 未来企业》(美)杰里·约拉姆·温德(Jerry Yoram Wind),(美)赫雷米·迈因(Jeremy Main)著;林洵子等译 1999
- 《Ant极限编程 利用JSP、EJB、XSLT、XDoclet和JUnit构建和部署Java应用程序》(美)Glenn Niemeyer,(美)Jeremy Poteet著;孟浩文译 2004
- 《超越预算 管理者如何跳出年度绩效评估的陷阱》(美)杰里米·霍普(Jeremy Hope),(美)罗宾·弗雷泽(Robin Fraser)著;胡金涛译 2005
- 《胆大为王 险中取胜的经营之道 being adventurous in life and work》(美)史蒂芬·卡特(Stephen Carter),(美)杰里米·克迪(Jeremy Kourdi)著;祝小静译 2006
- 《泰格·伍兹》Jeremy Roberts著 王乐译注 2006
- 《卫星通信 第2版》(美)Timothy Pratt,(美)Charles Bostian,(美)Jeremy Allnutt著;甘良才等译 2005
- 《甲壳虫乐队》Jeremy Roberts著 冯伟奇译注 2006
- 《道德与立法原理导论》(英)边沁(Jeremy Bentham)著;时殷弘译 2004
- 《简明大历史》(英)伊恩·克夫顿(Ian Crofton),(英)杰里米·布莱克(Jeremy Black) 2018
- 《英国政治通论》(英)奈杰尔·福尔曼,(英)道格拉斯·鲍德温著;苏淑民译 2015
- 《认识日本》(美)DuncanMcCargo著 2015
- 《树立成功的形象》(美)罗伯特·舒克著;康敬贻,熊国慧译 1989
- 《MACMILLAN DIRECTORY OF MULTINATIONALS VOLUME 2》D.C.STAFFORD 1989
- 《THE MACMILLAN COLLEGE HANDBOOK》GERALD LEVIN 1986
- 《THE MACMILLAN DICTIONARY OF ITALIAN LITERATURE》 1979
- 《THE MACMILLAN WRITER:RHETORIC》READER 1997
- 《MACMILLAN MODERN NOVELISTS MARK TWAIN》 1997
- 《THE MACMILLAN ENCYCLOPEDIA 1993 EDITION》 1993
- 《MACMILLAN ENCYCLOPEDIA OF CHEMISTRY VOLUME 3》 1997